서 론
비만은 전 세계적인 건강 및 경제적 문제로 부상하였으 며, 제2형 당뇨병, 암, 심혈관 질환 등 여러 질환과 관련이 있다. 비만 치료의 기본은 식이요법이나 신체활동 및 행동 수정이며, 보조적인 방법으로 항비만 약물을 사용하고 있지 만 비만의 유병률을 감소시키지 못하고 있는 실정이다. 더 불어 항비만 약물 중 대부분은 심혈관계 부작용, 자살위험 증가, 약물 의존 및 남용 가능성 등의 부작용과 관련이 있 다. 이러한 배경에서, 지방조직의 에너지 소비를 조절하여 비만의 근본적인 원인인 에너지 섭취와 소비의 불균형을 개선하는 것이 중요하다(Loh et al., 2017;Rivera & Chowen, 2019;Müller et al., 2022). 비만 및 대사질환의 또 다른 원인 으로는 지방세포에서의 미토콘드리아 기능장애가 있다. 미 토콘드리아는 진핵 생물에서 발견되는 세포소기관으로 에 너지 대사와 세포 항상성에 필수적이며, 지방세포의 분화 (지방생성)와 주요 기능에도 필요하다. Mitochondrial DNA (mtDNA) 양과 미토콘드리아 경로의 유전자 발현 수준 이 마른 쌍둥이에 비해 비만인 쌍둥이에서 하향 조절된다 는 것이 밝혀졌다(Pietiläinen et al., 2008;Boudina & Graham, 2014;Zheng et al., 2023). 따라서 비떨림 열발생(non-shivering thermogenesis)을 통한 에너지 소비의 촉진과 미토콘드리아 생합성(mitochondrial biogenesis) 및 미토콘드리아 기능 향상 이 비만 및 대사 관련 질환의 치료전략으로 관심을 받고있 다(Heinonen et al., 2020;Zhong et al., 2024).
지방조직은 기능과 형태에 따라 백색지방조직(white adipose tissue, WAT)과 갈색지방조직(brown adipose tissue, BAT)으로 나뉘고 백색지방은 트리글리세라이드 형태로 에 너지를 몸에 저장하며, 갈색지방은 수많은 미토콘드리아를 통해 열 형태로 에너지를 소비한다(Lizcano, 2019). 백색지방조직과 갈색지방조직의 중간 특성을 나타내는 베이지지방조직(beige adipose tissue)은 외부의 자극을 통해 백색지방조직의 browning 과정을 거쳐 갈색지방조직의 열발생 표현형을 갖게 된다. 이는 백색지방조직 저장소에서 열발생 관련 단백질의 발현을 상향조절하고 미토콘드리아의 밀도를 증가시켜 갈색지방조직의 특성을 나타내도록 유도한다(Alipoor et al., 2020;Flori et al., 2023). 비떨림 열발생은 추위 자극에 대한 반응으로 신체에서 열이 발생하는 것을 의미하며(Lettieri-Barbato, 2019), 포유동물의 경우 갈색지방조직에서 일어난다. 열발생 관련 주요 단백질 중 하나인 uncoupling protein 1 (UCP1)은 주로 갈색지방조직에 존재하며, 미토콘드리아 내막에 풍부하고 수소이온을 전기화학적 구배에 따라 미토콘드리아 기질로 운반시킴으로써 미토콘드리아 호흡의 에너지를 열로 방출시킨다(Coulson et al., 2021;Sanders et al., 2021). 따라서 백색지방의 과도한 축적으로 발생하는 비만 및 대사 관련 질환은 비떨림 열발생을 통해 불필요한 에너지를 소비함으로써 개선될 수 있다(Li et al., 2019).
인삼(Panax ginseng)은 두릅나무과(Araliaceae)에 속하는 다년생 초본식물로 동아시아, 중앙아시아, 북미 전역에 분포한다. 속명 Panax는 그리스어로 ‘모든 질병을 치료한다’는 뜻의 만병통치약(panacea)에서 유래되었다. 인삼은 오랜 시간 만병통치약, 장수촉진제로 여겨졌으며, 한국, 중국과 같은 아시아 국가에서 수많은 효능을 가진 강장제로 사용되어왔다(Nam et al., 2005;Im, 2020;Yu et al., 2024). Panax의 속은 17종으로 구성되어 있으며, 기능성 식품 및 의약품으로 가장 많이 사용되는 종으로는 Panax ginseng (인삼), Panax notoginseng (전칠삼), Panax quinquefolius (화기삼) 등이 있다(Mohanan et al., 2018). 인삼의 주요 활성성분인 진세노사이드(ginsenoside)는 화학구조에 따라 110여종 이상으로 분류된다(Ling et al., 2024). 뿐만 아니라 인삼에는 사포닌(saponin), 지방산(fatty acids), 다당류(polysaccharides), 미네랄 오일(mineral oils) 등 다양한 생리활성 단량체가 포함되어 있다(Shi et al., 2019). 이러한 다양한 생리활성 단량체에 의한 인삼의 항산화, 혈관성 치매 예방효과가 입증되었으며(Kim et al., 2011;Zhu et al., 2018) 인삼 뿌리에 다량 존재하는 천연 진세노사이드인 malonyl ginsenoside는 고지방식이와 streptozotocin으로 유발된 제 2형 당뇨병 쥐의 공복혈당을 감소시키며 인슐린 민감도를 개선시켜 항당뇨 효과가 입증되었다(Liu et al., 2013). 또한, 선행연구에 따르면 인삼 뿌리 추출물은 LPS가 처리된 RAW 264.7 세포에서 염증성 사이토카인인 IL-6, IL-1β의 mRNA 수준을 감소시키고 p62의 인산화를 증가시켜 autophagy를 유도함으로써 항염증 효과가 있다는 것이 밝혀졌다(Yang et al., 2022).
이처럼 인삼은 우수한 약리효과를 나타내는 것으로 알려져 있으나 비떨림 열발생과 미토콘드리아 생합성 및 그 기능에 연관된 연구는 부족한 실정이며, 인삼의 비떨림 열발생에 대한 체계적 문헌고찰과 메타분석은 시행된 바 없다. 따라서 본 체계적 문헌고찰과 메타분석을 통해 향후 비떨림 열발생에 관여하는 인삼의 효능에 대한 동물모델 실험의 기초자료로 활용하고자 한다.
재료 및 방법
체계적 문헌고찰을 위해 National Evidence-based healthcare Collaborating Agency (NECA)의 지침에 따라 핵심질문(PICO; participants, intervention, comparison, outcome)을 설정하였다. 연구대상은 인삼의 비떨림 열발생을 평가한 동물모델(rat, mouse)을 대상으로 하였으며 연령, 성별 등에는 제한을 두지 않았다. 중재는 인삼을 단일물질로 섭취하는 방식만 포함하였다. 연구 설계는 중재군과 대조군을 비교하는 동물실험 연구로만 제한하였다. 결과는 비떨림 열발생 관련 항목을 평가한 문헌을 모두 포함하였다.
국외 문헌 검색으로는 PubMed, EMBASE, Cochrane, Web of Science을 이용하였으며, 국내 문헌 검색으로는 한국학술정보(KISS), KoreaMed를 이용하였다. 사용된 검색어는 ‘Panax ginseng’ [MeSH Terms], ‘Ginsenosides’ [MeSH Terms]를 포함하도록 설정하였다. 문헌은 검색 일자인 2024년 4월 12일 기준으로 하였으며, 2010년 1월 1일부터 2024년 3월까지 출간된 문헌을 고찰하였다. 체계적 문헌고찰의 문헌 선정 기준은 1) 인삼에 관한 연구, 2) 비떨림 열발생에 관한 연구, 3) 동물실험(in vivo)에 관한 연구로 하였다. 배제 기준은 1) Panax ginseng이 아닌 다른 속명의 인삼에 관한 연구인 경우, 2) 인삼 또는 진세노사이드와 다른 물질을 복합 중재한 연구인 경우, 3) 전문을 확인할 수 없는 경우, 4) 이중게재가 확인된 경우에 배제하였다. 본 연구에서는 문헌 배제부터 이후 체계적 문헌고찰의 전 과정을 2명의 연구자가 각각 독립적으로 실시하였으며 의견이 일치되지 않는 경우 제 3자의 의견을 반영하여 최종 결정하였다. 1차 문헌 제목, 2차는 초록, 3차는 전문을 확인한 후 모든 기준에 부합하는 문헌을 최종 선정하였다. 문헌 배제와 중복 제거는 Microsoft Excel을 사용하였다.
선정된 7건의 문헌은 동물연구 비뚤림 위험 평가 도구인 SYRCLE (SYstematic Review Center for Laboratory animal Experimentation)의 risk of bias tool를 사용하였다. 평가 항목은 무작위 배정 순서(random sequence generation), 동물의 기저 상태(baseline characteristics), 배정은폐(allocation concealment), 무작위 거주(random housing), 연구자 눈가림 (blinding), 무작위 결과 평가(random outcome assessment), 평가자 눈가림(blinding of outcome assessment), 불완전한 결과자료(incomplete outcome data), 선택적 결과보고(selective reporting), 그 외 비뚤림(other bias), 전반적 편향(overall bias)으로 총 11가지 항목을 평가하였다. 위험 평가 결과는 ‘낮음(low risk)’, ‘일부 우려(some concerns)’, ‘높음(high risk)’으로 분류하였다.
메타분석 결과의 근거 신뢰도는 GRADE pro GDT tool (McMaster University and Evidence Prime Inc., Hamilton, ON, Canada)을 사용하여 비뚤림 위험(risk of bias), 비일관성(inconsistency), 비직접성(indirectness), 비정밀성(imprecision), 그 외 고려사항(others)으로 출판 비뚤림(publication bias)을 고려하여 총 5가지 기준으로 평가하였다. 5가지 기준을 모두 고려하여 근거수준 높은 경우 상향 평가되었으며, 근거 수준이 낮은 경우 하향 평가되었다. 전체적인 근거 수준 등급은 ‘높음(high)’, 보통(moderate)’, ‘낮음(low)’, ‘매우 낮음(very low)’으로 총 4단계로 분류하였다.
메타분석에는 Cocharne의 Review Manager (RevMan) Web을 사용하였다. 동물모델 특성상 높은 이질성이 있다고 판단하여 변량효과모형(random effect model)을 사용하였으며, 다양한 측정도구로 측정한 경우에 사용되는 연구결과를 동일한 단위로 표준화한, 표준화된 평균 차(standardized mean difference, SMD)와 95% 신뢰구간(confidence interval, CI)을 사용하였다. 또한 분석에 포함된 문헌들을 합성하여 메타분석 시행이 적절한지 판단하기 위해 Higgin의 I2 동질성 검정과 카이제곱 검정인 Q 통계량을 이용하여 이질성 검정을 시행하였다. 본 연구에 최종 선정된 7편의 문헌 중 중재군과 대조군의 결과변수를 종합하여 분석이 가능한 5편의 문헌으로 메타분석을 시행하였다. 결과변수 중 비떨림 열발생의 지표인 UCP1과 PGC-1α의 mRNA와 단백질 수준이 결과지표로 제시된 문헌을 구분하여 이에 대한 추정치를 분석에 활용하였다. Q 통계량을 제외한 분석에서 p<0.05인 경우 결과가 통계적으로 유의한 것으로 평가하였다.
결과 및 고찰
체계적 문헌고찰 대상 문헌은 선정 기준에 따라 검토 및 선발되었다(Fig. 1). 문헌검색 결과, PubMed 6,696건, EMBASE 3,615건, Cochrane 218건, Web of Science 8,488건, KISS 486건, KoreaMed 143건이 검색되었으며, Microsoft Excel로 중복 제거한 후 문헌의 수는 총 13,784건이었다. 선정 기준에 따라 문헌 제목과 초록 검토를 통해 13,773건의 문헌이 배제되었다. 전문 검토를 통해 전문을 확인할 수 없는 연구 1건, 비떨림 열발생과 관련이 없는 연구 2건, in vivo가 아닌 연구 1건이 배제되었다. 이를 통해 최종적으로 총 7건의 문헌이 선정되었으며, 최종 선정된 연구들의 각 특성을 요약하였다(Table 1). 이후 비뚤림 위험 및 근거 신뢰도를 평가하였다.
NO | Authors (yr) | Animal | Sex | Age (wk) | Weight (g) | N | Duration | Concentration (mg/kg) | Experimental groups | Results |
---|---|---|---|---|---|---|---|---|---|---|
|
||||||||||
1 | Chen (2017) | B61) | M | 5 | 18-20 | 10 | 12 wk | L: 125 | CON (LFD2)) | |
H: 500 | HFD3) | |||||||||
HFD+SLG4) (L) | ||||||||||
HFD+SLG (H) | ||||||||||
LFD+SLG (L) | ||||||||||
LFD+SLG (H) | ||||||||||
|
||||||||||
2 | Cho (2017) | SD rats21) | M | 6 | - | 8 | 4 wk | 200 | CON (normal saline) | |
WG22) | ||||||||||
SG-323) | ||||||||||
SG-624) | ||||||||||
SG-925) | ||||||||||
|
||||||||||
3 | Hong (2019) | B6 | M | 8 | 40 (HFD) | 6 | 10 days | 40 | chow | |
chow+Rb2 | ||||||||||
HFD | ||||||||||
HFD+Rb2 | ||||||||||
|
||||||||||
4 | Lim (2019) | B6, db/db mice | M | 6 | - | 5 | 6 wk | 10 | WT32) | |
db/db | ||||||||||
db/db+Rb1 | ||||||||||
|
||||||||||
5 | Lv (2023) | B6, ob/ob mice | M | 3 | - | 6 | 8 wk | 10 | ob/ob | |
PD33) (10 mg) | ||||||||||
PD+β2 | ||||||||||
receptor inhibitor (1 mg) | ||||||||||
|
||||||||||
6 | Meng (2023) | B6, ob/ob mice | M | - | 20± 2 | 5-8 | 5 wk | 10 | CON | |
25±2 | ob/ob | |||||||||
ob/ob+vehicle (DMSO39)) | ||||||||||
ob/ob+F1 | ||||||||||
|
||||||||||
7 | Yao (2020) | SPF43), B6 | M | 8 | 40 (HFD) | 10 | 23 days | 15 | CON (PBS44)) | |
1)B6: C57BL/6J mice, 2)LFD: low fat diet, 3)HFD: high fat diet, 4)SLG: stems and leaves of Panax ginseng, 5)FFA: free fatty acids, 6)TC: total cholesterol, 7)TG: triacylglycerol, 8)LDL-C: low-density lipoprotein cholesterol, 9)PPARγ: peroxisome proliferator-activated receptor gamma, 10)FAS: fatty acid synthase, 11)CD36: cluster of differentiation 36, 12)FATP2: fatty acid transport protein 2, 13)aP2: adipocyte protein 2, 14)HDL: high-density lipoprotein, 15)CPT-1: carnitine palmitoyltransferase-1, 16)UCP: uncoupling protein, 17)PPARα: peroxisome proliferator-activated receptor alpha, 18)HSL: hormone-sensitive lipase, 19)ATGL: adipose triglyceride lipase, 20)PGC-1α: peroxisome proliferator-activated receptor gamma coactivator 1-alpha, 21)SD rats: sprague-dawley rats, 22)WG: white ginseng, 23)SG-3: 3h-steamed ginseng, 24)SG-6: 6h-steamed ginseng, 25)SG-9: 9h-steamed ginseng, 26)IL-6: interleukin-6, 27)iNOS: inducible nitric oxide synthase, 28)iWAT: inguinal white adipose tissue, 29)eWAT: epididymal white adipose tissue, 30)BAT: brown adipose tissue, 31)p-AMPK: phosphorylated AMP-activated protein kinase, 32)WT: wild type, 33)PD: panaxadiol, 34)AUC: area under curve, 35)PRDM16: PR domain containing 16, 36)TFAM: transcription factor a mitochondrial, 37)p-CREB: phosphorylated cAMP response element-binding protein, 38)NRF1: nuclear respiratory factor 1, 39)DMSO: dimethyl sulfoxide, 40)gWAT: gonadal white adipose tissue, 41)NEFA: non-esterified fatty acids, 42)Cidea: cell death-inducing dna fragmentation factor alpha-like effector a, 43)SPF: specific-pathogen free C57BL/6J mice, 44)PBS: phosphate buffered saline, 45)Dio2: type II iodothyronine deiodinase
개별 문헌들의 연구 결과에 대한 체계적 오류를 검증하기 위해 비뚤림 위험 평가를 실시하였다(Fig. 2). 무작위 배정 순서(random sequence generation) 항목은 Chen et al. (2017), Lim et al. (2019), Yao et al. (2020), 그리고 Lv et al. (2023)의 연구가 비뚤림 위험 ‘낮음’으로 평가되었으며, Cho et al. (2017), Hong et al. (2019), 그리고 Meng et al. (2023)의 연구는 배정 순서에 관한 언급이 없어 비뚤림 위험 ‘일부 우려’ 로 평가하였다. 동물의 기저 상태(baseline characteristics) 항목에서는 Chen et al. (2017) 및 Yao et al. (2020)를 제외한 5편의 연구에서는 동물의 기저 상태에 대한 언급이 일부만 있어 비뚤림 위험 ‘일부 우려’로 평가하였으며, 2편은 동물모델의 연령, 최초 몸무게, 성별 등을 언급하여 비뚤림 위험 ‘낮음’으로 평가하였다. 배정은폐(allocation concealment) 항목은 7편 연구 모두 배정 순서 은폐와 관련된 내용을 언급하지 않아 비뚤림 위험 ‘일부 우려’로 평가하였다. 무작위 거주(random housing) 항목은 in vivo 에만 존재하고 각 실험군이 서식하는 곳의 환경이 균일하여 실험 결과에 영향을 주지 않는지를 평가하는 항목으로 온도, 습도 등 서식 환경을 언급한 Chen et al. (2017), Cho et al. (2017), Hong et al. (2019), 그리고 Meng et al. (2023)의 연구는 비뚤림 위험 ‘낮음’으로 평가하였으며, 이에 대하여 언급이 없었던 3편의 연구는 비뚤림 위험 ‘일부 우려’로 평가하였다. 연구자 눈가림(blinding), 무작위 결과 평가(random outcome assessment), 평가자 눈가림(blinding of outcome assessment) 항목은 7편 연구 모두 관련된 내용이 본문에서 확인되지 않아 비뚤림 위험 ‘일부 우려’로 평가하였다. 불완전한 결과자료(incomplete outcome data) 항목은 본문을 검토하였을 때 예상되는 모든 결과값을 보고한 것으로 판단되어 Cho et al. (2017) 및 Lim et al. (2019)는 비뚤림 위험 ‘낮음’으로 평가하였으며, 데이터에 대한 결측으로 판단되는 Chen et al. (2017)의 연구, 실험마다 n수가 상이하지만 이에 대한 언급이 없는 Yao et al. (2020)과 Lv et al. (2023)의 연구, n수의 범위가 넓은 Meng et al. (2023)의 연구는 비뚤림 위험 ‘높음’으로 평가하였다. 선택적 결과보고(selective reporting) 항목은 7편의 연구 검토 결과 연구방법에서 언급한 평가 도구들을 모두 보고한 것으로 판단되어 비뚤림 위험 ‘낮음’으로 평가하였다. 그 외 비뚤림(other bias) 항목은 Chen et al. (2017), Cho et al. (2017), 그리고 Hong et al. (2019)의 연구는 그 외 비뚤림 항목이 존재한다고 판단하기 어려워 ‘일부 우려’로 평가하였으며, Lim et al. (2019), Yao et al. (2020), Lv et al. (2023), 그리고 Meng et al. (2023)의 연구에서는 각 실험마다 동물모델 또는 중재방법이 상이한 것으로 판단되어 비뚤림 위험 ‘높음’으로 평가하였다. 모든 평가 결과를 종합하여 전반적 인 편향(overall bias) 항목을 평가한 결과, Cho et al. (2017)와 Hong et al. (2019)의 연구를 제외한 5편의 연구가 비뚤림 위험 ‘높음’으로 평가되었다.
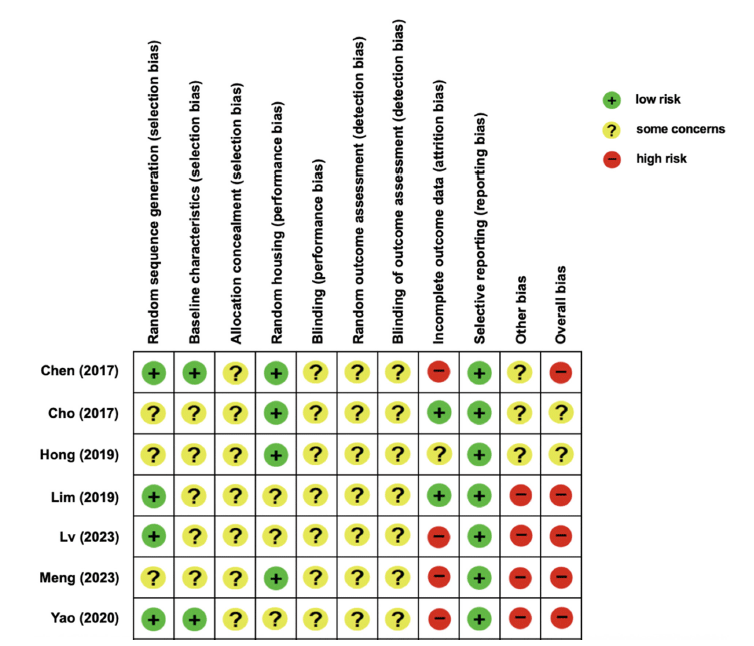
근거 수준 평가는 메타분석 시 이용된 지표에 대해 진행하였다(Table 2). 비일관성은 이질성 결과에 따라 평가되었으며, 비직접성은 해당 근거가 연구결과에 미치는 영향으로 판단되었다. 비정밀성은 95% 신뢰구간(95% CI)을 통해 평가되었으며 그 외 고려사항은 출판 비뚤림에 의해 평가되었다. 출판 비뚤림은 연구가 출판될 때 유의미한 결과를 나타내는 연구가 그렇지 않은 연구보다 출판될 가능성이 더 높기 때문에 편향이 야기될 수 있음을 의미한다(Begg & Berlin, 1988). 전반적인 근거수준을 평가한 결과 UCP1 mRNA (Chen et al., 2017;Hong et al., 2019;Meng et al., 2023)의 근거수준은 ‘매우 낮음’으로 평가되었다. UCP1 단백질(Lim et al., 2019;Lv et al., 2023;Meng et al., 2023)의 근거수준은 ‘높음’으로 평가되었다. PGC-1α mRNA (Chen et al., 2017;Hong et al., 2019;Meng et al., 2023)의 근거수준은 ‘보통’으로 평가되었다.
메타분석 시행의 적절성을 평가하는 이질성 검정의 경우, I2의 범위에 따라 해석이 된다. 대략적으로 0-25%인 경우 ‘이질성 낮음’, 25-50%인 경우 ‘중간 정도의 이질성이 있음’, 50-75%인 경우 ‘상당한 이질성이 있음’, 75% 이상인 경우 ‘높은 이질성이 있음’을 의미한다(Cochrane training, 2022). Q 통계량은 각 연구들의 중재 효과가 공통 중재 효과추정치로부터 얼마나 멀리 떨어져 있는지를 검정하는 방법이며, I2보다 연구의 수에 민감한 특징이 있기 때문에 낮은 검정력을 가질 수 있다는 점을 고려하여 p<0.10인 경우 결과가 통계적으로 유의한 것으로 평가한다(Kang, 2015).
미토콘드리아 내막에 위치한 UCP1은 갈색지방조직의 중요한 지표이며, uncoupling 효과로 인한 browning의 가장 직접적이고 중요한 매개체이다(Xiong et al., 2021). UCP1은 추위 노출이 부족하여 교감신경계가 활성화되지 않은 생리학적 조건에서는 비활성 상태이지만(Li & Fromme, 2022), 추위 노출 및 스트레스 등의 생리적 자극을 받게 되면 β-아드레날린 수용체(β-adrenergic receptor, β-AR) 및 하류 신호 전달 경로가 촉진되어 활성을 띠게 된다. 이후 미토콘드리아 내막을 가로질러 양성자 누출을 시작하며 이는 포도당과 지방에서 유래된 환원된 기질의 이화작용을 실질적으로 증가시킨다(Okamatsu-Ogura et al., 2020;Mills et al., 2022). 실험조건이 실험군마다 상이하게 설정되어 메타분석에서 제외된 Yao et al. (2020) 연구에 따르면 비만이 유도된 쥐에게 인삼(ginsenoside Rd)을 급여하였을 때 UCP1의 mRNA 수준의 증가가 실온에서는 유의적인 결과를 나타내지 않았지만 4°C 즉, 추위에 노출시켰을 때는 UCP1의 mRNA 수준이 대조군과 비교하여 약 5배 정도 증가한 것을 확인했다. UCP1은 전자전달 사슬에서 생성된 미토콘드리아 양성자 운동력을 소산시키며 설치류와 인간 뿐만 아니라 변온성 척추동물과 정온성 포유류에서도 발현되어 열발생을 넘어 생물학에서도 중요한 역할을 한다(Jastroch et al., 2005;Lidell et al., 2013;Oelkrug et al., 2014). 이와 관련된 선행연구에 따르면, UCP1이 결핍된 마우스에서 갈색지방조직의 미토콘드리아는 활성산소종(reactive oxygen species, ROS) 유도 기능장애에 더 민감하고 칼슘 완충능력이 손상되어 세포사멸과 관련된 선천 면역경로 조절에 영향을 미친다는 것이 보고되었다(Kazak et al., 2017). 메타분석을 진행한 5편의 문헌 중 3편의 문헌에서 중재군의 UCP1 mRNA 수준이 음성대조군과 비교하여 통계적으로 유의하지 않았으며, 중간 정도의 이질성을 나타내었다(SMD = 1.11; 95% CI: -0.21~2.43; p = 0.10, I2 = 47%) (Fig. 3A). 반면, 3편의 문헌에서 중재군의 UCP1 단백질 수준은 음성대조군과 비교하여 통계적으로 유의하게 높았으며, 이질성은 없는 것으로 확인하였다(SMD = 1.26; 95% CI: 0.46-2.07; p = 0.002, I2 = 0%) (Fig. 3B). Meng et al. (2023)의 문헌은 UCP1의 mRNA 및 단백질 수준이 모두 측정되어 두 개의 분석에 모두 포함되었다.
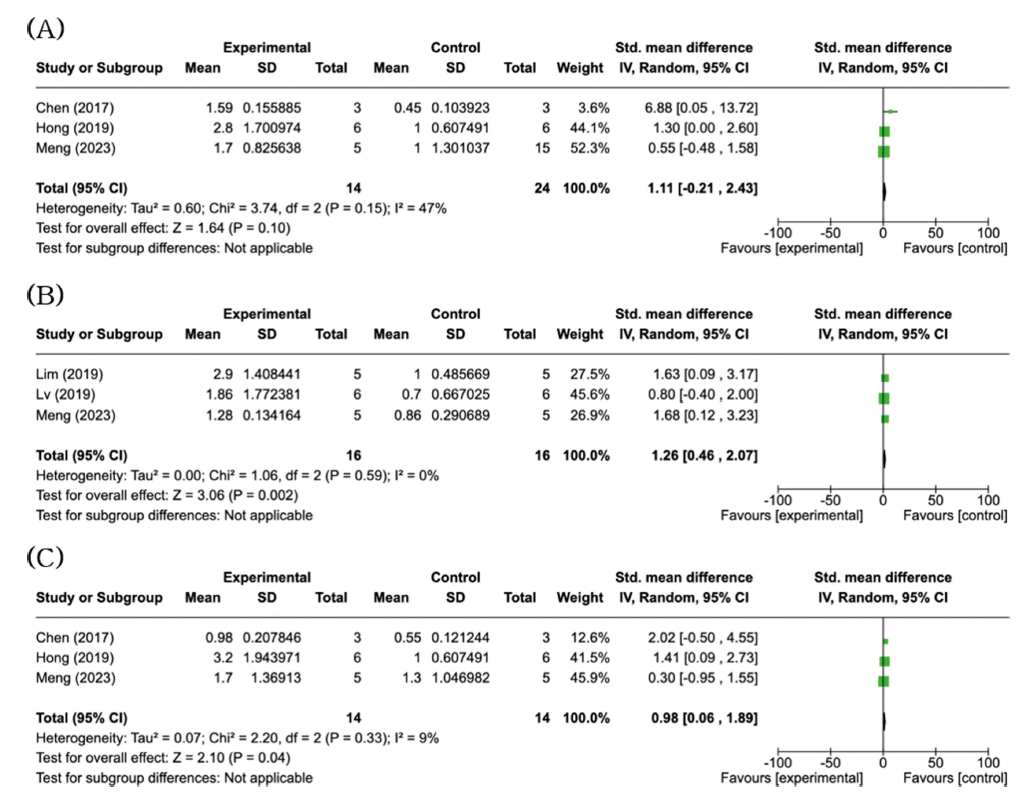
Peroxisome proliferator-activated receptor gamma coactivator 1-alpha (PGC-1α)는 미토콘드리아 생합성 및 세포 에너지학의 주요 전사 공동활성자로서, 분화된 갈색지방세포(brown adipocyte) 및 베이지지방세포(beige adipocyte)에서 다양한 전사조절자와 상호작용하여 열발생 유전자 활성을 조절하는데 중심적인 역할을 한다(Inagaki et al., 2016). PGC-1α는 UCP1 조절 DNA 요소에 결합된 peroxisome proliferator-activated receptor gamma (PPARγ) 및 갑상선 호르몬 수용체(thyroid hormone receptor)와 같은 핵 수용체를 공동 활성화하여 UCP1 유전자의 전사를 활성화시킨다(Lidell et al., 2014). 선행연구에 따르면, PGC-1α에 대한 지방 특이적인 기능이 제거된 대립유전자를 갖는 마우스에서 지방 PGC-1α의 손실은 피하지방에서 미토콘드리아, 지방산 산화 및 열발생 유전자 발현을 감소시키는 것으로 보고되었다(Kleiner et al., 2012). PGC-1α가 제거된 마우스에 의해 PGC-1α가 갈색지방세포 분화의 분자적 측면에서 필요하지 않지만 갈색지방세포의 분화 동안 미토콘드리아 수와 기능의 감소에 기여하기에 열발생 유전자 발현에는 PGC-1α가 필수적이라는 것이 밝혀졌다(Uldry et al., 2006). 또한, PGC-1α가 결핍된 마우스는 추위에 대한 방어능력이 크게 감소하여 저체온증으로 사망할 수 있으며, 지방산 산화 및 미토콘드리아 기능에 관여하는 많은 유전자의 mRNA 수준이 낮아졌다(Lin et al., 2004). Lv et al. (2023)의 연구에서는 고지방식이를 급여한 마우스와 비교하여 인삼(PD)을 급여한 마우스의 지방조직에서 PGC-1α의 단백질 수준이 유의적으로 증가하였다. 메타분석 결과, 5편의 문헌 중 3편의 문헌에서 중재군의 PGC-1α mRNA 수준이 음성대조군과 비교하여 통계적으로 유의하게 높았으며, 이질성이 낮았다(SMD = 0.98; 95% CI: 0.06-1.89; p = 0.04; I2 = 9%) (Fig. 3C).
메타분석 결과를 종합적으로 평가하였을 때, 동물모델에서 인삼에 의한 UCP1의 mRNA 수준의 유의적인 증가는 관찰되지 않았다. 하지만, UCP1의 단백질 수준은 유의적으로 증가하였으며, PGC-1α의 mRNA 수준도 유의적으로 증가하는 결과를 나타냈다. 따라서 인삼이 비떨림 열발생 관련 지표들의 발현을 증가시킬 수 있다고 판단된다. 비교분석 기준을 충족하지 못해 메타분석에서는 제외되었지만 비떨림 열발생에 관여하는 인자인 PR domain containing 16 (PRDM16)과 미토콘드리아 기능에 관여하는 mitochondrial transcription factor A (TFAM)의 단백질 및 mRNA 수준의 유의적인 증가가 일부 문헌에서 관찰되었다.
갈색 및 베이지지방세포 결정 및 분화과정에 중요한 조절자인 PRDM16 (Hussain et al., 2020)은 CCAAT/enhancer-binding protein beta (CEBPβ), PPARγ, peroxisome proliferator-activated receptor alpha (PPARα) 및 PGC-1α에 결합하고 이를 공동조절하여 갈색지방세포 특이적인 유전자 유도를 촉진한다(Genchi et al., 2023). 선행연구에서, 지방세포 분화에 영향을 미치는 PPARγ가 유전적으로 없는 상태에서 PRDM16에 의해 UCP1과 PGC-1α를 포함한 여러 갈색지방조직 유전자의 발현이 유도되었다(Seale et al., 2007). PRDM16의 지방 특이적 기능의 결핍은 베이지 지방세포의 열발생 기능을 크게 감소시키며, 마우스의 피하지방조직에서는 염증 유전자의 발현과 대식세포의 축적이 증가하였다(Cohen et al., 2014). Hong et al. (2019)에 따르면 유의적이지는 않으나 인삼(Rb2)을 투여한 군에서 mRNA 수준이 증가하는 경향을 나타냈으며, Lv et al. (2023)의 연구에서는 인삼(PD)을 급여한 군에서 고지방식이를 급여한 군에 비해 유의적으로 PRDM16의 단백질 수준이 증가했다. 또한 Yao et al. (2020)의 연구에서 고지방식이를 급여한 마우스에게 15일 동안 인삼(ginsenoside Rd)을 투여했을 때 갈색지방조직에서 PRDM16의 mRNA 수준이 대조군과 비교하여 유의적으로 증가하였다. 최종 선정된 7편의 문헌 중 3편의 문헌을 통해 PRDM16의 단백질 및 mRNA 수준이 인삼투여에 따라 증가했음을 확인하였다.
TFAM은 mtDNA의 전사를 촉진시켜 미토콘드리아 생합성을 증가시키며 TFAM에 의해 증가된 미토콘드리아는 더 많은 UCP1을 발현시키므로 열발생과 관련이 있다(Jeremic et al., 2017). mtDNA는 응축된 DNA-단백질 복합체에 존재하는 이중 가닥 원형 DNA 분자로, 미토콘드리아 기능은 mtDNA에 극도로 의존적이다. mtDNA의 안정성 유지는 주로 mtDNA 복제 및 전사에 필요한 TFAM에 의해 매개된다(Zhao et al., 2021). PGC-1α는 nuclear respiratory factor 1 (NRF1)에 대한 공동 활성화제 역할을 하여 TFAM의 발현을 유도한다(Tabuchi & Sul, 2021). TFAM 동형접합성 형질전환 마우스의 갈색지방세포는 human TFAM (hTFAM)을 과발현하여 산화적 인산화와 미토콘드리아 기능을 증가시키며, 세포 외 소포 분비를 촉진하여 갈색지방세포를 더 활성화시킨다(Fujii et al., 2022). TFAM의 지방 특이적 감소를 갖는 마우스는 산화적 인산화 기능 장애와 백색지방조직에서 세포사멸 및 염증을 유도하였으며 갈색지방조직이 하얗게 변하는 특성이 발견되었다(Vernochet et al., 2014). 또 다른 선행연구에서는 갈색지방조직 특이적 TFAM 결핍 마우스가 mtDNA로 인코딩된 전자전달계 유전자의 발현 감소와 갈색지방조직의 호흡결함을 나타냈다(Masand et al., 2018). 본 연구의 문헌에서는 Lv et al. (2023)의 연구에서 인삼(PD)을 급여한 군에서 고지방식이를 급여한 군에 비해 유의적으로 TFAM의 단백질 수준이 증가하였다.
이 외에도 열 발생에 관여하는 type II iodothyronine deiodinase (Dio2)는 Yao et al. (2020)의 연구에서, cell death-inducing DNA fragmentation factor alpha-like effector A (Cidea)는 Yao et al. (2020)과 Meng et al. (2023) 2개의 연구에서 측정되었다. 고지방식이를 급여한 마우스에게 15일 동안 인삼(ginsenoside Rd)을 투여하고 4°C에 노출시켰을 때, 대조군 대비하여 Dio2와 Cidea의 mRNA 발현이 유의하게 증가하는 것을 확인하였다. 추위에 노출되는 동안 교감신경 자극이 주된 역할을 하게 되며 이 때, 갑상선 호르몬인 thyroxine (T4)을 3,5,3′-triiodothyronine (T3)으로 전환시키는 효소인 Dio2를 유도하여 세포 내 T3가 증가되어 갈색지방조직이 활성화된다(Yau & Yen, 2020). 선행연구에 따르면, Dio2가 손상되면 지방세포 분화와 UCP1, PGC-1α의 발현이 감소되는 것으로 밝혀졌다(Hall et al., 2010). 또한, Cidea는 지방세포에서 지질 방울의 형성과 축적을 조절하며, 스트레스 상황에서 세포 사멸을 유도하는 기능을 한다(Slayton et al., 2019). 인간 지방세포의 browning 동안 Cidea는 농도 의존적인 비정상적 핵 이중 신호를 통해 지질 방울에서 핵으로 이동되며, 핵에서 PPARγ의 UCP1 증강 인자 결합을 강화하여 UCP1 전사를 유도한다(Jash et al., 2019). 따라서 잘 알려진 전사인자인 UCP1, PGC-1α 뿐만 아니라 PRDM16, TFAM, Dio2, Cidea와 같은 지표들이 인삼의 미토콘드리아 기능 향상 및 비떨림 열발생과 관련이 있다고 판단되지만 이에 관해서는 추가적인 연구가 필요하다.
요 약
본 연구는 인삼을 동물모델에게 급여하였을 때, 비떨림 열발생에 미치는 영향을 파악하기 위해 해당 주제로 출판된 문헌에 대해 체계적 문헌고찰 및 메타분석을 실시하였다. 총 7편의 문헌들의 결과를 종합하였을 때, 동물모델에서 인삼은 열발생과 미토콘드리아의 생합성을 증가시키는 유전자의 발현을 촉진하여 비떨림 열발생을 유도할 수 있을 것으로 판단된다. 또한, 인삼이 미토콘드리아의 수 증가 및 그 기능을 향상시켜 비떨림 열발생을 촉진하는 것으로 사료되나 관련 연구가 부족하여 이에 관해서는 추후 연구가 필요하다. 본 체계적 문헌고찰과 메타분석이 갖는 한계는 제한된 비떨림 열발생 관련 지표로 인해 최종 선정된 7편의 문헌의 공통된 결과지표가 부족하여 5편의 문헌으로 메타분석이 실시되었다는 점, 각 논문의 비뚤림 위험성이 대부분 ‘높음’이였다는 점, 최종 선정된 7개의 문헌 중 유의적인 결과가 존재하지 않는 1편의 문헌이 포함되어 있다는 점이다. 또한 해당 주제의 연구 표본의 수가 적고, 메타분석에 사용된 결과수치가 각 연구의 본문에 정확한 값으로 서술되지 않아 결과 그래프에 의존하여 연구자들의 판단에 의한 추정치로 메타분석이 실시된 점을 들어 본 연구가 제시하는 분석 결과를 해석하는 데 주의가 필요하다.