Introduction
Vegetable seed oils (VSOs) have been extracted and used not only as ingredients in food and as sources of dietary lipids, but also as sources of nutraceuticals used to overcome the various oxidative stresses that contribute to the development of diseases, including cancer, cardiovascular ailment, and various chronic conditions (Concepcion et al., 1998; Wagner et al., 2000; DO, 2002; Bianchi et al., 2003; Marina et al., 2009). Many chemical and enzymatic changes occur in food, including off-flavoring, oxidative rancidity and hydrolysis; generally, VSOs are more susceptible to these oxidative changes than animal fats are due to their containing more unsaturated fatty acids. Various factors accelerate these chemical changes, such as air content, heat, light and the presence of trace metals and moisture (Wagner et al., 2000; DO, 2002; Lee et al., 2004; Yoon et al., 2008), leading to a loss of health benefits (Hasmadi et al., 2005). Several methods exist to inhibit this effect, including vacuum packaging, modified atmosphere packaging, refrigerating and freezing; overall, though, the addition of antioxidants is the most powerful way to retard this reaction (Lee et al., 2004).
Plants seeds generally contain a high number of these antioxidants, potentially making them resistant to this oxidative effect. Perilla seed and flaxseed oil are both primarily composed of α-linolenic acid, making them an important source of ω-3 fatty acids (Kurowska et al., 2003; Lee et al., 2004; Marina et al., 2009). Pine seed oil, on the other hand, is mainly comprised of linoleic acid, making it an ω-6 fatty acid source. Meanwhile, camellia seed and olive oil contain high amounts of oleic acid, which could make them particularly susceptible to oxidative rancidity (Bianchi et al., 2003; Mateos et al., 2005; Long et al., 2008). However, olive oil is also particularly rich in antioxidant polyphenols and flavonoids (Mateos et al., 2005; Josiah et al., 2016).
In order to better investigate the effect of varying unsaturated fatty acid composition on oxidative stability, this study aimed to compare acid composition for various VSOs and to determine stability while accounting for different storage conditions.
Materials and Method
Perilla, camellia and pine seeds were purchased from a local market (Agricultural Marketing Center, Seoul, Korea), while olives and flaxseeds were purchased from an Agricultural Market (Tokyo, Japan).
Vegetable seeds were roasted in an electric roaster (EL-24, Dongbang Machine Co., Daejeon, Korea) for 15 min under constant stirring and at temperatures up to 190 °C; this roasting temperature was commonly used because it provided a high yield and a desirable flavor. The roasted seeds were then immediately moved into a mechanical press (DB-3000, Dongbang Machine Co., Daejeon, Korea) and pressed at 700 kgf/cm2 for 10 min to obtain the VSOs. Moisture, crude fat, crude protein and ash composition were then determined using the AOAC method (AOAC, 1980).
The VSOs (5 g) were saponified by refluxing in 500 mL of 1.25 N NaOH solution in 95% ethanol for 30 min. After the solution was cooled down, 200 mL of 2 N HCl was added and the solution was mixed vigorously. The mixture was extracted twice with 500 mL hexane, which was then washed with water until neutral pH was achieved. The hexane layer was dried over Na2SO4, after which fatty acids were recovered through rotary evaporation and subjected purging with N2. 4 mL of BF3-methanol was added to the fatty acids to yield fatty acids methyl esters which were extracted with n-hexane. Then, 1 μL aliquots of the extracts were injected into a gas chromatograph (GC) (Varian 3800, Varian, Walnut Creek, CA, USA) equipped with a flame ionization detector and a fused silica capillary Supelcowax-10 GC column (30 m × 0.32 mm i.d.; Supelco, Bellefonte, PA, USA). The injector, oven and detector temperatures were 240, 190 and 260°C, respectively. Fatty acid analysis was conducted in triplicate for each sample; each trial was conducted separately from the others. VSO fatty acid composition was determined using a GC (Agilent 6890 Plus, Agilent, Palo Alto, CA, USA) equipped with a fused silica capillary supelcowax-10 GC column and coupled to a mass spectrometer (5973N GC-MSD, Agilent, Palo Alto, CA, USA) equipment with Mass Lab. Helium was used as the carrier gas which was set to a flow rate of 1 mL/min. The injector temperature was set to 250 °C; samples were injected under the same conditions as reported in earlier work (Heliana de Azevedo et al., 2003). Spectra were recorded at an electron energy of 70 eV and an ion source temperature of 270 °C, and were compared with available data in mass spectra libraries.
First, multiple 50 g samples of each VSO were transferred to their own glass bottles and stored at 30, 37 or 45 °C for 35 d. Samples at each temperature were left open to O2 exposure, sealed to prevent O2 exposure, sealed and stored with O2 scavenger; O2-Zero (TPG, Gimpo, Korea). All samples were stored in the dark. Aliquots from each sample were extracted after shaking the respective bottles and were analysed for peroxide value (POV) (Hasmadi et al., 2005), p-anisidine value (p-AnV) (Marina et al., 2009), iodine value (IV) and thiobarbituric acid (TBA) value after 1, 3, 7, 9, 12, 15, 18, 25, 28 and 33 d. Every measurement was performed three times.
POV was determined according to an established AOCS International procedure (Hasmadi et al., 2005). The sample (3 g) was weighed in a 250 mL glass Erlenmeyer flask equipped with a stopper and was then thoroughly agitated for 3 min in 30 mL of an acetic acid-chloroform solution (3:2, v/v) until total dissolution was achieved. The samples were then vacuum filtered to remove the VSOs. Saturated potassium iodide solution (0.5 mL) was added to the filtrate, which was then transferred into the burette of an automatic titrator equipped with a stirrer. The sample was titrated against a standard solution of sodium thiosulfate (25 g/L). POV is calculated and expressed in milliequivalents (meq) of peroxide per kg of oil which was calculated according to the following formula:
where, S is titration of sample and B is titration of blank solution.
The extent of saturation of fatty acid samples was measured using IV (AOCS, 1990). The sample (0.1- 0.3 g) was weighed in a flask then was thoroughly agitated for 3 min in a solution composed of 10 mL chloroform and 25 mL Wijs solution until total dissolution. An excess of iodine monochloride contained in the Wijs solution was allowed to react with the double bonds in the sample, after which any unreacted halide was titrated with 10% sodium thiosulfate. IV is therefore calculated and expressed as reacted iodine per g of sample.
where, V0 is titration of blank solution and V1 is titration sample.
Formation of secondary oxidation products was measured by p-AnV according to a previously published procedure (Marina, 2009). The sample (0.5 g) was weighed in a flask and was thoroughly agitated for 10 min in a solution composed of 5 mL iso-octane and 5 mL p-anisidine until total dissolution. Absorbance at 350 nm was then measured using UV-Vis spectrophotometry (Optizen POP, Mecasys Co. Ltd., Daejeon, Korea). The p-AnV was calculated using the following equation:
where, As is the absorbance the value of the sample with the p-anisidine solution and Ab is the absorbance the value of the sample without the p-anisidine solution.
TBA value was determined according to a previously published procedure of AOAC method (AOAC, 1980). The sample (3 g) was mixed with 10 mL benzene and 2 mL TBA reagent (2-thiobarbituric acid 0.69 g + H2O 100 mL + acetic acid 100 mL); the resulting solution was then shaken for 4 min. After agitation 2 mL of the separate at bottom was added to 2 mL TBA solution in a test tube. The test tubes were incubated at room temperature in the dark for 30 min, after which absorbance at 530 nm was measured using UV-Vis spectrophotometry (Optizen POP, Mecasys Co. Ltd., Daejeon, Korea).
where, A is the absorbance the value of the sample and B is the absorbance the value of the blank solution.
Each reported value is the mean of the three samples prepared for each test. The data were analysed by ANOVA and Duncan’s multiple range tests. Statistical significance was accepted at a level of p < 0.05 (Lee et al., 1998).
Results and Discussion
Table 1 shows the proximate composition of all test vegetable seeds. Each showed over 60% crude lipid content, and 15% crude protein content, and 3% of moisture content. The high concentration of crude lipids and proteins may be the source for various functional activities of vegetable seeds.
Table 2 shows the fatty acid composition of the tested VSOs, demonstrating fairly typical distribution. No differences in fatty acid compositions have been observed between corn, soybean, sesame and perilla seed oil in the past (Kurowska et al., 2003; Marina et al., 2009). The major fatty acid present in camellia seed and olive oil was oleic acid, at fatty acid concentration of 84.7 and 82.0 %, respectively, values that were in good agreement with previous results (Bianchi et al., 2003; Mateos et al., 2005; Qing et al., 2014). Perilla seed and flaxseed oil were notably composed of linolenic acid, at concentration of 45.5 and 59.7%, respectively, whereas pine seed oil showed linoleic acid in the highest concentration, at 48.3%. Camellia seed and olive oil were the least unsaturated, with extent of unsaturation values of 95.0 and 94.0, respectively, while flaxseed oil showed the highest value of 223.3. The extent of unsaturation correlates well with oxidative stability results from previous research (Lee et al., 2012). This is consistent with the notion that increased unsaturation increases susceptibility to oxidation.
According to POV tests, VSO oxidative stability decreased with increasing storage temperature and time. After 35 d, all samples showed similar POVs with respect to each other given the same storage conditions (Fig. 1), with the POV rate constants increasing as a function of temperature for all oils regardless of storage conditions (Fig. 2). However, the extent of change for those samples exposed to O2 was significantly greater than for those that were not, indicating that O2 plays a larger role overall. For example, the POV rate constant for the flaxseed oil sample containing the O2 scavenger was about 4 times greater than that of the sample that simply sealed at 45 °C.
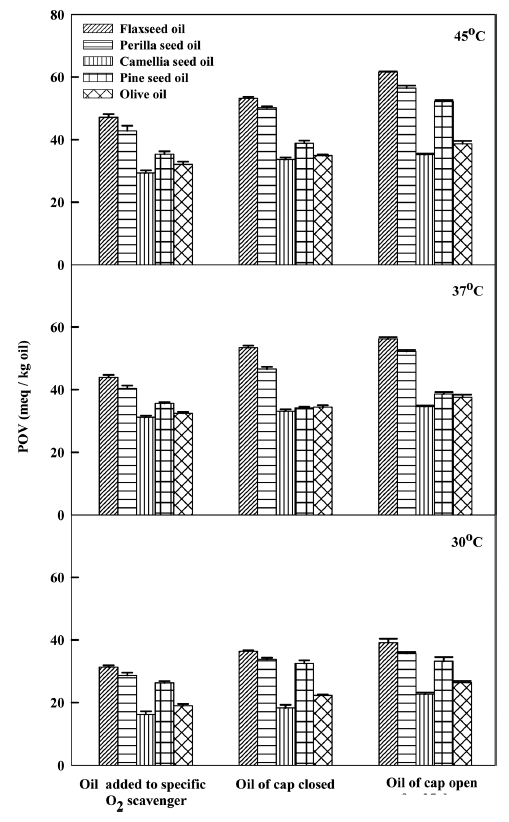
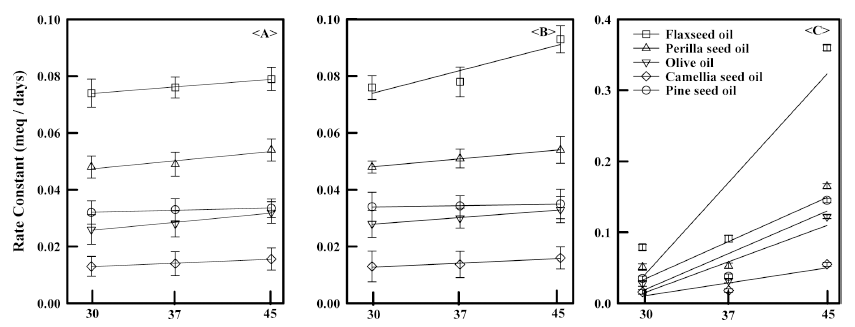
Overall, camellia seed oil showed the lowest POV rate constant for all storage conditions, while flaxseed oil showed the highest. All VSOs showed their highest POVs at 45 °C when exposed to O2. Similar results were mentioned (Lee et al., 1998; Shahina et al., 2005) for sesame seed, perilla seed, and olive oil (Yang et al., 1996; Valerity et al., 2016). Again, note that the degradation results are consistent with what would be expected given the extent of unsaturation of each oil.
IV tests are useful in that they indicate the extent to which the double bonds in the fatty acids are oxidized by iodine; generally, minimal change in IV for changes in temperature or storage conditions indicates increased stability. Overall, the IVs of the VSOs decreased, as temperature increased (Table 3). Generally, oxidation consists of a complex series of chemical reactions characterized by the formation of free radicals and a decrease in unsaturation due to hydrogen abstraction at the adjacent double bond. The observed decrease should therefore have been expected seeing how temperature reduces activation energy and increases molecular mobility. The control values that were obtained before storing the VSOs were in good agreement with the extent of unsaturation for each oil, suggesting a direct link between IV and fatty acid composition. Flaxseed, perilla seed and pine seed oil all showed a dramatic increase at 45 °C, a pattern that mirrors the POV data. However, pine seed oil showed a dramatic increase even at 37 °C under O2 exposure, a change that was not mirrored for POV. Again, IV rate constants were more affected by O2 than temperature; the lowest IV rate change was observed in samples at low temperature that were not exposed to O2, which likely resulted from removal of residual O2 by the O2 scavenger and the decreased molecular mobility of the oils (Yang et al., 1996).
1) Mean±SD (n=3); a-c Superscriptive letters in a row indicate a significant difference at p<0.05 by Duncan's multiple comparison.
p-AnV can serve as an indicator for oxidative stability, with higher p-AnV indicating more products from oxidative processes; again, minimal change in this value when changing temperature or storage conditions is an indicator of increased stability. As shown in Table 4, this measure of oxidative stability provided results that were in line with both the other chemical tests and the fatty acid composition profile of the VSOs. Notably, by product formation in flaxseed oil stored at 45 °C and exposed to O2 was almost 1.5 times higher than that in the corresponding pine seed oil sample and 3 times higher than that in camellia seed oil.
1) Mean ± Standard deviation (n=3) ; a-c Superscriptive letters in a row indicate a significant difference at p<0.05 by Duncan's multiple comparison.
Overall, p-AnV changes were less affected by storage conditions, unlike the other test results. For example, the difference p-AnV between flaxseed oil samples stored at 45 °C and exposed to oxygen and stored at 30 °C and containing an O2 scavenger was only about 8%; the corresponding differences in IV and POV rate constants were 14.7% and 80.0%, respectively. Notably, camellia seed oil formed the fewest by products when compared to the control, while flaxseed oil showed the highest; this observation was in good agreement with both the other test results and previous reports (Yang et al., 1996; Kim et al., 2003; Papadimitriou et al., 2006).
As shown in Table 5 and Fig. 3, flaxseed and perilla seed oil showed the highest change in TBA values, while olive and camellia seed oil showed the least with a difference of nearly 2 times between them for samples stored at 45 °C and exposed to O2. TBA values are frequently employed to track oil quality during storage as well as to assess products that had been stored for an unknown amount of time. In this assay, TBA reacts with malonaldehyde, a byproduct of lipid oxidation, to form a red complex which can be tracked by spectrophotometer. Higher values therefore reliably indicate less oxidative stability. Note that the TBA results are therefore in very good agreement with other tests. TBA values and rates were more affected by oil type, storage time and temperature than the other assays, an observation that is consistent with previous researches (Yang et al., 1996; Kim et al., 2003; Shahina et al., 2005).
1) Means±SD (n=3); a-cSuperscriptive letters in a row indicate a significant difference at p<0.05 by Duncan's multiple comparison.
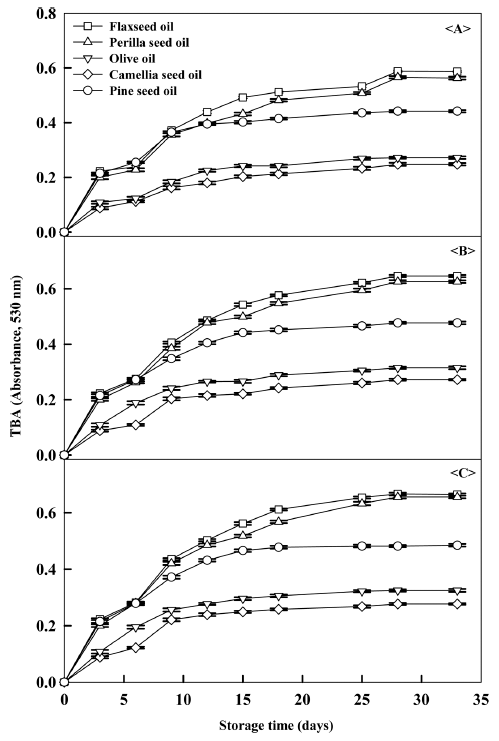
Overall, oxidative stability generally decreased for increasing unsaturated fatty acid content. However, when oleic acid was the dominant unsaturated fatty acid, oxidation was inhibited. Oleic acid content may therefore be used as an indicator for the oxidative susceptibility of lipid during processing.