Introduction
Yogurt, a dairy product known for centuries, is produced by fermenting milk with two prevalent bacteria, Lactobacillus bulgaricus and Streptococcus thermophilus. These bacteria transform lactose in milk into lactic acid, reducing the pH, and generating a range of volatile and non-volatile compounds, including exopolysaccharides. These processes lend yogurt its distinctive tangy flavor and thick, creamy texture (Lee & Lucey, 2010).
Yogurt, an excellent source of protein, is also rich in essential minerals such as calcium, phosphorus, and potassium. Furthermore, it provides a beneficial quantity of probiotics, live bacteria that can optimize digestive metabolism by restoring the microbiota populations within the digestive tract (Aryana & Olson, 2017). Numerous studies suggest that regular yogurt consumption may help decrease the risk of diabetes, obesity, and metabolic syndrome (Ejtahed et al., 2011;Sayon-Orea et al., 2017). Innovation in yogurt products has flourished over time, leading to a vast array of diverse yogurt-based offerings. Ongoing advancements and modifications aim to continually augment yogurt’s health benefits (Aryana & Olson, 2017). The fortification of yogurt with fruits and vegetables introduces prebiotic fibers (Allgeyer et al., 2010) and not only enriches the product’s color and flavor (Smith & Hui, 2004) but also enhances its phytochemical antioxidant content (Sarker et al., 2022). Noni (Morinda citrifolia), belongs to Rubiaceae Family, is an indigenous plant in Southeast Asia, Australia, South America, and Africa. Historically, it has been recognized for its medicinal attributes, purportedly possessing anti-inflammatory and antioxidant properties (Wang et al., 2002). The noni fruit boasts a wealth of vitamins and minerals, including vitamin C, potassium, and calcium. A diverse array of 160 phytochemical compounds has been identified within the fruit, encompassing organic acids, multiple anthraquinones (such as damnacanthal), phenolic compounds, and alkaloids (Ali et al., 2016). As such, the noni fruit might reduce inflammation and protect against free radical damage. Furthermore, there is suggestive evidence in some research that the noni fruit may exhibit anti-cancer characteristics (Chan-Blanco et al., 2006). As noted by Wang et al. (2002), NJ has been traditionally consumed as a therapeutic alternative for many illnesses, including arthritis, diabetes, hypertension, headaches, AIDS, cancer, and peptic ulcers.
Noni fruit, recognized for its significant nutrient profile, is frequently used for juice extraction and consumed worldwide as a health-enhancing beverage. Distinct extraction methods yield two variants of NJ: traditional and non-traditional, along with other formulations such as Noni beverage, Noni squash, and Noni syrup. The traditional extraction method involves fermenting the fruits in airtight containers for at least two months at room temperature, followed by juice extraction, filtration of impurities, and bottling. The bottled juice is stored at ambient temperature or refrigerated to prolong its shelf life (Wang et al., 2002). Significant compounds have been identified in the noni fruit, including phenolics like damnacanthal and scopoletin, organic acids such as caproic and caprylic acid, vitamins including ascorbic acid and provitamin A, and amino acids such as aspartic acid, in addition to various minerals (Chan-Blanco et al., 2006).
The symbiotic properties of combining foods such as yogurt and fruit have yet to be explored. Both food groups are nutrient-dense and have shown protective associations against specific diet-related diseases (DRDs) in epidemiological studies. The potential for the synergistic effects of yogurt and fruit consumption on DRDs and their synbiotic potential as foods with probiotic and prebiotic properties was explored (Fernandez & Marette, 2017;Yadav et al., 2022). Therefore, these bioactive compounds in NJ may be leveraged to augment the health advantages of cow’s milk. A fusion of NJ and yogurt is anticipated to yield a nutritionally balanced food by enhancing the functional attributes of yogurt. However, NJ may influence the yogurt’s rheological properties, sensory and microbial characteristics, and shelf-life (Ahmad et al., 2022).
The quality of yogurt is affected by many factors during the fermentation process, including the concentration of the added compound, incubation time and temperature, and the use of starter cultures. These variables can significantly affect the pH, acidity, Brix, syneresis, and the nutritional and microbial properties of the yogurt (Lee & Lucey, 2010;Aldaw et al., 2019;Krisnaningsih et al., 2019). Within the framework of this study, we explore the impacts of the quantity of NJ incorporated, the incubation time, and the temperature on both the physicochemical, lactic acid bacterial and sensory attributes of yogurt fortified with NJ. Additionally, we aim to discern the optimal levels of these factors to augment the acceptance and marketability of this functional yogurt.
Materials and Methods
Utra-High Temperature (UHT) fresh milk-plain, sweetened condensed milk (Vinamilk, Ho Chi Minh City, Vietnam), and NJ (Bestone, Quang Nam Province, Vietnam) were purchased from local supermarkets in Da Nang City, Vietnam. The starter culture powder for yogurt fermentation (Yogourmet, Lallemand, Canada) contains Lactobacillus bulgaricus, Streptococcus thermophilus, Lactobacillus casei, and Bifidobacterium longum.
A mixture design containing several formulations (Table 1) was used to evaluate the effect of NJ. The yogurt formulation consisted of cow milk, NJ, and a starter culture inoculum. In this study, the cow milk was a mixture of sweetened condensed milk and UHT milk in a ratio of 1:6 (v/v), as derived from our previous research (Tran & Nguyen, 2023).
Ingredients | Treatments | |||
---|---|---|---|---|
|
||||
C0 | NJ1 | NJ3 | NJ5 | |
|
||||
Cow milk (mL) | 100 | 99 | 97 | 95 |
NJ (mL) | 0 | 1 | 3 | 5 |
Inoculum (%, w/v) | 0.5 | 0.5 | 0.5 | 0.5 |
The cow milk was initially pasteurized at 90-95°C for 10 min to eliminate any pathogenic microorganisms. Subsequent to this, all samples were fortified with NJ before fermentation. The mixture was rapidly cooled to 38-46°C and inoculated with 0.5% (w/v) of Yogourment starter bacterial culture powder. The mixture was then incubated at variable temperatures as per the specifications of the experimental design. Post-fermentation, the yogurts were preserved in a refrigerator and maintained at 4°C until further analysis. The entire fermentation process was executed in triplicate to ensure the reproducibility of the results.
NJ was analyzed for moisture, protein, ash and carbohydrates according to AOAC standard method (AOAC, 1990).
NJ and yogurt samples were chemically analyzed for Brix (Total Soluble Solids TSS), titratable acidity (TA) and pH. TSS was measured with a hand refractometer (AOAC, 1990). The sample’s TA was determined by titration against 0.1 N NaOH and expressed as a percentage of lactic acid. The pH value of the samples was measured during fermentation and storage using a pH meter.
The TPC in NJ or yogurt samples was measured using a modified protocol of the Folin-Ciocalteu method (Song et al., 2010). Initially, 1 g of yogurt was diluted with 7 mL of distilled water at 25°C, then mixed on a shaker at 120 rpm for 30 min, and cooled to 5°C. Afterward, the mixture was centrifuged at 5,000 rpm for 5 min, and the supernatant was filtered through Whatman No. 1 paper and stored at -20°C.
For TPC analysis, 0.5 mL of the NJ or yogurt extract was combined with 0.5 mL of distilled water and 1 mL of Folin-Ciocalteu reagent (diluted 1:10, v/v). The mixture was left to incubate in the dark at room temperature for 5 min, then 1 mL of 10% (w/v) sodium carbonate solution was added. It was further incubated in the dark for 30 min. Absorbance was then measured at 760 nm using a spectrophotometer. TPC was determined using a gallic acid standard curve and expressed as mg gallic acid equivalents (GAE) per 100 mL.
The physical stability of yogurt was assessed by determining syneresis using the Deshpande et al. (2019) method. A wire mesh screen was placed over a funnel in a graduated cylinder, and 25 g of yogurt was placed on it. After being weighed, the samples were immediately refrigerated at 6°C. After 2 h of refrigeration, the volume of the collected exudate was measured and reported as syneresis (mL/100 g).
The total count of LAB was conducted using the pour plate method. To prepare the MRS agar, 55.2 g of MRS powder was mixed with 1 L of distilled water and autoclaved. After cooling the agar to 45°C, 15 mL was poured into a petri dish and left at room temperature to solidify. Next, 0.1 mL of diluted yogurt was transferred to the MRS agar and spread evenly using a sterilized spreader. The plates were then inverted and incubated at 37°C for 48 h. The LAB count was calculated using the formula: CFU/mL = (Total colonies formed x dilution factor) / 0.1 mL.
Sensory analysis was conducted to evaluate differences among yogurt samples. A 5-point hedonic scale of TCVN 10565-3:2015 was used to rate the samples on specific indicators, including texture, color, flavor, and taste. Twenty-one semi-trained panelists from the faculties and students of the Faculty of Chemical Technology-Environment, University of Technology and Education, University of Da Nang, Vietnam assessed the sensory quality of yogurts. Panelists rated each yogurt sample on a scale of 1 (bad) to 5 (excellent) using the words “poor” (2), “fair” (3), and “good” (4). Individual booths were used for sensory evaluations to avoid score bias. Yogurt samples were served in paper cups marked with three-digit codes and randomized among and within assessors. The panelists used water to cleanse their palates between samples (TCVN, 2015).
Data on physical and sensory characteristics were analyzed using ANOVA, with a significance level of 5%, to identify differences in the fermentation process of NJ-fortified yogurt. The groups were compared using Fisher’s test to observe significant differences. Stargraphics Centurion XVI was used to process all data. Microsoft Excel 2019 was used for graphic illustration.
Results and Discussion
The properties of NJ are recorded and presented in Table 2.
NJ contains 94.07% water. The primary components of its dry matter are total solids (7.1oBx), carbohydrates (3.43%) and proteins (2.36%). The ash content is 0.59%, which may be low due to the juice-making processes. The NJ was extracted, and the residue was discarded.
This NJ, obtained after the traditional fermentation process of noni fruit, has an acidic nature (titratable acidity = 1.95%) and a relatively low pH (pH = 3.44). Therefore, integrating it into yogurt can be challenging due to its high acid content, potentially leading to casein micelle denaturation and viscosity changes. Moreover, NJ has a brown color, a wine-like aroma, and a slightly bitter, strong taste. Consequently, yogurt with NJ will have different sensory characteristics, such as structure, aroma, and taste, compared to yogurt made entirely from animal milk.
The results of NJ were in close agreement with a previous study of Chan-Blanco et al. (2006). Deshpande et al. (2019) found that NJ had a moisture content of 95.93%, TSS of 8.6%, and carbohydrates of 3.45%, with a pH of 3.62. However, they reported lower protein and ash content (only 0.43% and 0.23% respectively). The differences in NJ composition could be due to variations in planting materials, soil, climate conditions in each country, or the fermentation process to make NJ.
TPC is a vital indicator of the antioxidant capacity of foods. Phenolic compounds, a diverse group of secondary metabolites found abundantly in plants, exhibit significant biological activities. These include antioxidant capacity, hepatoprotective effects, antimicrobial properties, anti-inflammatory benefits, and hypotensive activity (Sang et al., 2001;Chan-Blanco et al., 2006;Samoylenko et al., 2006). Noni fruit is rich in more than 150 phytochemicals, with phenolics being the most prevalent (Valdés et al., 2009). Phenolic compounds can scavenge reactive oxygen species due to their electron-donating properties. This ability allows them to protect cells against oxidative damage, potentially preventing chronic diseases such as cancer, cardiovascular disease, and diabetes (Wang & Su, 2001;Yang et al., 2007a;Yu et al., 2008;Ali et al., 2016). Therefore, foods with high TPC are considered beneficial for health due to their potential to mitigate oxidative stress and reduce the risk of chronic diseases.
In this study, the TPC of NJ was found to be 262.20±21.84 mg GAE/100 mL. Literature reports on the TPC of NJ vary widely, with values ranging from 3.1 to 210 mg GAE/100 mL (Yang et al., 2007a;Yang et al., 2007b;Prasetyo et al., 2018). Although the Folin-Ciocalteu colorimetric assay is a standard method for determining phenolic content in beverages, TPC results can vary significantly based on sample preparation parameters, such as extraction solvents and incubation times. The TPC value of 262.20±21.84 mg GAE/100 mL found in this study suggests that NJ is a powerful functional ingredient that can enhance the health-promoting properties of fortified foods.
NJ was found to have an acidic nature with a low pH and unusual sensory characteristics. Therefore, the physicochemical properties and LAB of the yogurt samples were studied to evaluate the effectiveness of the process and the suitability of the concentrated NJ (Table 3).
The values indicate that the pH of the fruit juice-fortified yogurt samples decreases as their acidity increases. This could be due to an accelerated rate of fermentation and the presence of lactic acid-producing organisms. NJ contains a variety of micronutrients, non-volatile and volatile components, ketones, lactones, beta-carotenoids, terpenoids, and proxeronine (Ali et al., 2016). Key compounds identified in noni fruit include phenolics like damnacanthal and scopoletin, organic acids such as caproic and caprylic acid, vitamins like ascorbic acid and provitamin A, amino acids including aspartic acid, and various minerals (Chan-Blanco et al., 2006). These organic acids, vitamins, amino acids, and minerals present in NJ enhance the fermentation process by supplying essential nutrients and boosting the metabolic activity of LAB. Moreover, the phenolic compounds in NJ help prevent spoilage by inhibiting the growth of spoilage microorganisms such as yeasts and molds during fermentation (Chan-Blanco et al., 2006). The combined effect of these components enhanced fermentation activity and a quicker reduction in pH. These findings align with previous research on incorporating plant extracts into yogurt. Plant extracts are known for their health-promoting properties, such as antimicrobial and antioxidant effects, and can serve as valuable sources of functional nutrients (Joung et al., 2016). For example, moringa leaves are rich in phenols, proteins, minerals, and vitamins, and provide substantial phytonutrients (Saini et al., 2016). Adding moringa extract has been shown to accelerate yogurt fermentation compared to control samples, as reported by Zhang et al. (2018). Similarly, herbal extracts and essential oils from dill (Anethum graveolens), peppermint (Mentha piperita), and basil (Ocimum basilicum) have demonstrated significant antioxidant (Lu & Yeap Foo, 2001) and antimicrobial activity (Marino et al., 2001). These properties contribute to faster pH reduction rates in herbal-yogurts than plain-yogurts (Amirdivani & Baba, 2011). Additionally, green tea is recognized for its health benefits, including an antimicrobial effect that does not harm LAB. The addition of green tea powder to yogurt has also been shown to expedite the decrease in pH, compared to plain yogurt (Jeong et al., 2018).
Syneresis, also known as whey separation, is a critical quality indicator of yogurt. This defect in yogurt production can negatively impact its shelf life and aesthetic appeal. A higher syneresis value implies lower yogurt quality (Kiros et al., 2016). Our results showed that increasing amounts of NJ up to the NJ3 samples significantly reduced syneresis. However, syneresis significantly increased in NJ5 yogurts compared to NJ3. Sarker et al. (2022) reported that adding 30% carrot juice to yogurt resulted in the lowest syneresis, while higher concentrations increased it (Sarker et al., 2022). Adding fruit or fruit juice decreases the water-holding capacity of protein in yogurt and decreases the water-holding capacity of protein in yogurt, which may cause an increase in syneresis (Cakmakci et al., 2014). Furthermore, the TSS of NJ-fortified yogurt samples decreased as the concentration of added juice increased. This result is similar to Deshpande et al. (2019). The acidity of fortified yogurt samples increased as the total solids content decreased, possibly due to the increased moisture availability for starter activity or carbohydrates. Reducing sugar can accelerate acid metabolism in yogurt (Deshpande et al., 2019).
The TPC in yogurt fortified with NJ significantly increased with greater NJ fortification. In particular, the control sample with no NJ had a TPC of 14.07±2.19 mg GAE/100 mL. As NJ fortification rose to 1%, 3%, and 5% (NJ1, NJ3, and NJ5), the TPC correspondingly increased to 24.79±3.61 mg GAE/100 mL, 36.97±2.72 mg GAE/100 mL, and 45.60±1.78 mg GAE/100 mL, respectively. This illustrates a clear positive correlation between the quantity of added NJ and the phenolic content of the yogurt.
These results align with previous research highlighting the abundance of phenolic compounds in fruit juice. Liu & Lv (2019) found that the polyphenol content rose with the addition of more blueberry flower pulp. Sarker et al. (2022) reported a similar rise in TPC with increased carrot juice fortification in yogurt.
In contrast to the TPC trend, the concentration of LAB slightly declined with higher levels of NJ fortification. The control had an LAB count of 2.03 × 109 CFU/mL. The LAB counts for NJ1, NJ3, and NJ5 were 1.42 × 109 CFU/mL, 1.28 × 109 CFU/mL, and 1.27 × 109 CFU/mL, respectively. Despite this decrease, statistical analysis revealed no significant difference between these values, indicating that the reduction is not statistically significant.
The minor decrease in LAB concentration with greater NJ fortification could stem from the antimicrobial properties of phenolic compounds in NJ. Phenolic compounds may disrupt microbial cell membranes and inhibit enzyme activity (Bouarab-Chibane et al., 2019;Ecevit et al., 2022). However, the fortification levels used in this study did not significantly impact LAB viability, indicating that the yogurt matrix might partially mitigate these effects.
In conclusion, acidity increased while pH and TSS decreased as more NJ was added. Syneresis decreased until 3% NJ was added to the yogurt, then significantly increased with more NJ. Fortifying yogurt with NJ significantly increases its TPC, enhancing its potential health benefits due to its high antioxidant capacity. The NJ does not significantly affect the viability of LAB, ensuring the yogurt’s probiotic properties are maintained despite a slight LAB reduction. Thus, an optimal concentration of 3% NJ could be beneficial for yogurt fortification.
The research examined how adding NJ to yogurt affects its sensory qualities. The average sensory scores for NJ-fortified yogurts are presented in Fig. 1.
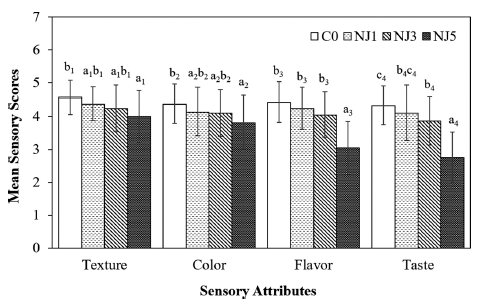
A semi-trained panel evaluated the juice-fortified yogurts, focusing on organoleptic aspects such as texture, color, flavor, and taste.
As the concentration of NJ increased, the yogurts’ sensory scores decreased. Yogurts with lower NJ concentrations were preferred over those with higher concentrations. However, the addition of NJ3 resulted in only a slight decline in sensory attributes, such as texture, color, and flavor, compared to the NJ1 and the C0. In contrast, NJ5 significantly reduced all sensory attributes. The decline is likely due to NJ components affecting the yogurts’ firmness, cohesiveness, color, and flavor profiles.
Taste scores for NJ3, while lower than the control, did not significantly differ from NJ1, suggesting the flavor profile at higher NJ concentrations may be less appealing, possibly due to acidity and bitterness from NJ compounds, likely contributing to a less favorable taste profile. Despite the slight decline in sensory scores for NJ3 compared to NJ1 and C0, NJ3’s scores for texture (4.24), color (4.10), aroma (4.05), and taste (3.86) remain within an acceptable range. This indicates that NJ3 maintains a good balance of desirable sensory qualities.
Considering physicochemical properties and LAB content as the above results, NJ3 offers enhanced antioxidant activity and maintains probiotic qualities. Therefore, NJ3 is optimal for NJ-fortified yogurt, balancing health benefits with sensory acceptability. These results align with Deshpande et al. (2019), who found that adding 3% noni fruit juice to yogurt enhances its sensory properties.
The incubation time and temperature can significantly influence the acidification process and the characteristics of the final yogurt product (Jumah et al., 2001;Lucey, 2004;Lee & Lucey, 2010;Loveday et al., 2013;Krisnaningsih et al., 2019). This paper evaluates the impact of incubation time and temperature on yogurt’s physicochemical properties and LAB with added NJ, focusing on parameters such as pH, TSS, titratable acidity, syneresis, TPC, and LAB (Table 4).
The pH values significantly decreased with longer incubation times and higher temperatures. The incubation temperature and time directly influence the pH values of the yogurt. The pH reduction can be attributed to the high bacterial metabolic activity, which consumes lactose and produces lactic acid. The incubation temperature and duration profoundly impact microbial metabolism and the subsequent lactic acid fermentation. Using a higher incubation temperature and longer incubation time led to a decline in pH values (Lee & Lucey, 2010;Medeiros et al., 2015). The point at which the pH reaches 4.6 is often considered the end of yogurt fermentation (Kristo et al., 2003). This study demonstrates that after a 10-hour incubation period at 42°C, the pH of yogurt supplemented with NJ attains the isoelectric point of casein (pH 4.6).
The samples became increasingly acidic as the incubation temperature and time increased. Consequently, the higher the incubation temperature and time, the greater the acidity of yogurt added with NJ. The escalating total acidity trend can be attributed to the accumulation of a variety of organic and acetic acids, products of the fermentation activity carried out by lactic bacteria (Oladimeji et al., 2016;Krisnaningsih et al., 2019).
Brix values, indicating TSS content, showed a significant increase with longer incubation times, from 13.53% at 8 h to 15.07% at 12 h. These findings can be reasoned with the extended incubation period, which potentially intensified the fermentation process and produced some by-products after ending fermentation at pH 4.6 (Lee & Lucey, 2010). Although temperature-wise, Brix values significantly decreased at 42°C (13.67%), compared to 14.63% at 38°C and 14.67% at 46°C. This observed decrease can be attributed to the utilization of soluble solids for bacterial metabolism, with carbohydrates serving as an energy source for LAB growth and metabolic activities. This is corroborated by similar findings in a study where the carbohydrate content exhibited a reduction from 5.01% at 18 h of incubation to 3.35% after 42 h of incubation (Krisnaningsih et al., 2019).
Syneresis is an important phenomenon; its properties can diminish the product’s shelf-life and acceptability. The contraction of a gel without any external force application, known as spontaneous syneresis, typically results in whey separation (Lucey, 2004;Lee & Lucey, 2010). The syneresis of yogurts is significantly influenced by incubation time and temperature. At 8 h, syneresis was 3.20%, decreasing to 2.83% at 10 h, but increasing to 3.70% at 12 h. At 38°C, syneresis was significantly higher (13.23%) compared to 2.83% at 42°C and 5.17% at 46°C. The initial reduction followed by an increase suggests optimal gelation occurs at 10 h and 42°C, beyond which whey separation becomes more pronounced.
The TPC of the yogurt increased significantly with longer incubation times, from 27.81 mg GAE/100 mL at 8 h to 37.92 mg GAE/100 mL at 10 h, then significantly decreased to 31.25 mg GAE/100 mL at 12 h. At 38°C, TPC was 55.74 mg GAE/100 mL, decreasing to 34.57 mg GAE/100 mL at 42°C and 25.19 mg GAE/100 mL at 46°C. The results suggest that the increase in TPC at 10 h is due to the effective release of phenolic compounds, facilitated by microbial activity and the breakdown of complex structures in NJ (Takó et al., 2020). The subsequent decrease at longer incubation times and higher temperatures arelikely due to microbial utilization, polymerization, and binding of phenolics, as well as non-thermal degradation processes (Liyana-Pathirana & Shahidi 2005;Abad-García et al., 2007;Uma et al., 2010;Mokrani & Madani 2016).
LAB counts varied with both incubation time and temperature. The highest LAB count was observed at 10 h (2.74 × 109 CFU/mL) compared to 1.79 × 109 CFU/mL at 8 h and 1.83 × 109 CFU/mL at 12 h. Temperature-wise, LAB counts were relatively stable at 38°C (2.51 × 109 CFU/mL) and 42°C (2.44 × 109 CFU/mL) but decreased significantly at 46°C (1.63 × 109 CFU/mL). These findings indicate that moderate temperatures and optimal incubation times favor LAB growth, while extreme conditions may inhibit viability (Adamberg et al., 2003). Medeiros et al. (2015) highlighted the significant impact of incubation temperature on the yogurt fermentation process. This temperature also affects microbial metabolism and the subsequent lactic acid fermentation. The incubation temperature, a crucial factor, influences the metabolic activity of enzymes. These enzymes exhibit optimum performance at a specific temperature, at which enzymatic reactions are accelerated to their maximum rate (Wardani et al., 2017).
The study demonstrates that both incubation time and temperature significantly affect the physicochemical properties of NJ-fortified yogurt. Optimal conditions (10 h at 42°C) yield the best balance of low syneresis, high TPC, and viable LAB counts, enhancing the yogurt’s functional and health-promoting properties.
As previously mentioned, the incubation time and temperature significantly affect the yogurt-making process and the final product’s features, impacting all of the yogurt’s sensory attributes. These sensory properties, including texture, color, flavor, and taste, are crucial in determining consumer acceptance and overall product quality. The provided data shows significant changes in these sensory attributes with different incubation times (Fig. 2a) or temperatures (Fig. 2b).
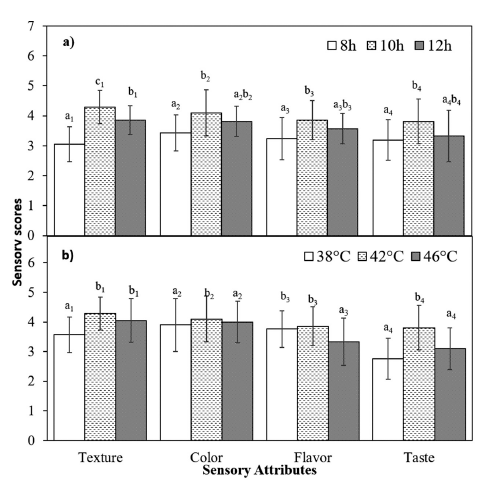
The sensory evaluation showed that texture, color, flavor, and taste all improved significantly at an incubation period of 10 h and a temperature of 42°C. These conditions resulted in the best sensory attributes, likely due to optimal fermentation, balanced acid production, and ideal gel formation (Oladimeji et al., 2016;Krisnaningsih et al., 2019). However, a slight decline in scores at 12 h or a significant decrease in most sensory scores at 46°C suggests potential over-fermentation, leading to less optimal sensory qualities. Other studies have also shown that as the incubation temperature increases, certain sensory attributes, including mouth coating and the smoothness of stirred yogurts, decline (Martin et al., 1999).
In conclusion, the optimal fermentation conditions for NJ-fortified yogurt are 10 h of incubation at 42°C, balancing physicochemical properties and sensory attributes to produce a high-quality product.
Conclusion
The research determined that adding 3% Bestone NJ and incubating for 10 h at 42°C with the starter culture powder Yogourmet (comprising Lactobacillus bulgaricus, Streptococcus thermophilus, Lactobacillus casei, and Bifidobacterium longum) produces the best NJ-fortified yogurt characteristics. This formulation achieves superior physicochemical and sensory qualities and enhances the yogurt’s health-promoting properties, making it a functional food with significant antioxidant and probiotic benefits.
The enhanced TPC indicates an increase in antioxidant properties, which can contribute to improved health outcomes by neutralizing free radicals in the body. The high LAB counts ensure that the yogurt maintains its probiotic benefits, supporting gut health and potentially improving the immune response. The balance between TPC and LAB is crucial for the overall functionality of the yogurt, making it a well-rounded functional food.
Future studies should optimize fermentation and storage conditions to improve NJ-fortified yogurt quality and shelf-life. Key areas include understanding TPC and LAB stability, their interaction with yogurt components, and maintaining health benefits. Sensory property research during long-term storage can enhance consumer acceptability and product appeal.