Introduction
A hangover is an unpleasant adverse reaction that is both mental and physical, caused by drinking excessive alcoholic beverages (Swift & Davidson, 1998). Typically, a hangover occurs within several hours after the cessation of drinking, coinciding with a decrease in blood alcohol concentration (BAC) (Dilley et al., 2018). The most common symptoms of an alcohol-induced hangover include thirst, sleepiness, headache, sensitivity to light and sound, fatigue, depression, anxiety, and increased blood pressure (Piasecki et al., 2017;van Schrojenstein Lantman et al., 2017). When alcohol is ingested, it travels to the stomach; a small amount is immediately absorbed through the oral mucosa and esophagus, while the remaining alcohol enters the stomach and upper part of the small intestine, where it is absorbed through diffusion (Stermer, 2002;Maccioni et al., 2023). Absorbed alcohol is distributed through the blood to various tissues of the body, including the brain and liver, and some of it is excreted through the lungs, urine, and sweat without being metabolized (de la Monte et al., 2009;Cederbaum, 2012;Shin et al., 2020).
Metabolism is a chemical reaction occurring in the cells of living organisms, transforming ingested substances into metabolites. In some cases, metabolites can become more toxic than the original substance, causing harm to body tissues. Alcohol is primarily degraded through alcohol dehydrogenase pathways, with 80-90% metabolized by ADH in liver cells (Edenberg, 2007;Jelski & Szmitkowski, 2008;Jelski et al., 2008b). Alcohol is first metabolized to acetaldehyde, an intermediate product, which is then further metabolized to acetate. Cytochrome P450 and catalase also play roles in decomposing ethanol into acetaldehyde alongside ADH. The isozyme CYP2E1 of cytochrome P450 is a crucial pathway for alcohol oxidation, especially activated during chronic alcohol exposure or elevated BAC (Doody et al., 2017;Jiang et al., 2020;Contreras-Zentella et al., 2022). This metabolic process occurs in the endoplasmic reticulum and is vital for handling increased alcohol levels (Ji, 2012, 2014).
Metabolism of alcohol by CYP2E1 enhances reactivity in neutrophils and macrophages of the liver, brain, heart, lungs, and immune system, generating acetaldehyde and reactive oxygen species (ROS) (Linhart et al., 2014;García-Suástegui et al., 2017;Xu et al., 2017). Acetaldehyde and ROS are highly reactive and toxic, potentially causing tissue damage (Wu and Cederbaum, 2003;Novitskiy et al., 2006). While Class I ADH primarily exists in the liver, catalase, found in intracellular structures such as peroxisomes in various organs and tissues, also oxidizes alcohol using hydrogen peroxide (H2O2) to convert it to acetaldehyde, though the amount decomposed is minimal (Jelski et al., 2008a;Contreras-Zentella et al., 2022;Lu & George, 2024).
Asians generally have difficulty metabolizing acetaldehyde efficiently, leading to the toxic effects of unoxidized acetaldehyde and alcohol, which damage liver and brain cells and reduce metabolic rates (Jayawardena et al., 2017;Martinez-Hurtado et al., 2018). Hangover severity can vary based on individual health factors such as gender and age, and chronic alcohol consumption can pose significant social and economic issues, necessitating effective management (White, 2020;Abebe et al., 2021;Vatsalya et al., 2023). Morning Care (MC) is one of the hangover drink products for hangover-relieving after alcohol consumption in Korean culture. This study aimed to investigate the efficacy of hangover remedies focused on enzymes involved in alcohol metabolism to alleviate hangover symptoms following alcohol consumption.
Material and Methods
Morning Care H (1996018809268), Morning Care PRESSON H (20060445110852), Morning Care PRESSON G (20060445110853) and Morning Care EX (20080181270179) were provided from Dong-A Pharm. Each product includes a number assigned by the regulatory authority when a food manufacturing or processing business reports the production of an item. The detailed list of ingredients for each product is shown in Table 1.
The C57BL/6J mice, both male and female, weighing 16-18 grams at 6 weeks old, were acquired from ORIENT BIO Inc. in Seongnam, Republic of Korea. They were housed in a cage with controlled parameters, including 23±2°C, 55±10% relative humidity, and 12-h light/dark cycles. The mice were given access to commercial feed from ORIENT BIO Inc. and tap water on demand for a week. In order to create an alcohol-induced hangover model, mice were given 20% v/v ethanol through oral gavage for 30 min while also receiving multiple MC products (20 mL/kg b.w.). Mouse was placed into five groups (n = 12 per group) after a period of acclimation. Group 1 received water administration and ethanol feeding; group 2 received Morning Care H from Dong-A Pharm company administration and ethanol feeding; group 3 received Morning Care PRESSON H administration and ethanol feeding; group 4 received pro Morning Care PRESSON G administration and ethanol feeding; and group 5 received Morning Care EX administration and ethanol feeding. The samples were given to each group 30 min either before or after the ethanol feeding. One, three, or five hours following ethanol feeding, the mouse’s blood and liver were removed. The Hanyang University Institutional Animal Care and Use Committee examined and approved all animal experimentation protocols (protocol 2023-0127), which were carried out in compliance with Korean Food and Drug Administration regulations.
Mouse blood was extracted into 500 μL, centrifuged at 3,500×g for 10 min, and then kept at -80°C until analysis. The Ethanol Bio HT (Roche, Darmstadt, Germany) was used to measure the amount of alcohol present in serum.
The activity of ADH and ALDH in mouse liver was measured using an alcohol dehydrogenase activity assay kit and an aldehyde dehydrogenase activity colorimetric assay kit (Sigma-Aldrich Co.). After adding 200 μL of either ADH or ALDH buffer to 100 μg of mouse liver, the tissues were destroyed by ultrasonography (using a vibrating cell from SONICS, Newtown, CT, USA) for 5 sec, then centrifuged at 13,000×g for 10 min. To measure the activity of ALDH and ADH, the supernatant was utilized. The amount of enzyme needed to produce 1.0 μmole of NADH per minute at pH 8.0, 37°C (for ADH) or room temperature (for ALDH), is known as one unit of ADH or ALDH.
A sonicator was used to destroy 2 mg of liver tissue, and the RNeasy Mini Kit (QIAGEN, Hilden, Germany) was used to extract the RNA in order to evaluate the expression level of the ADH and ALDH genes in mouse liver. The FastStart Essential DNA Green Master (Roche) was utilized as the master mix for real-time PCR after the Transcriptor First Strand cDNA Synthesis Kit (Roche, Basel, Switzerland) was utilized to generate the cDNA. The following primers were created by Jung et al. (2007): GAPDH (housekeeping gene, 5′-ATC CCA TCA CCA TCT TCC AG-3′; forward primer, 5′-CCT GCT TCA CCA CCT TCT TG-3′; reverse primer), ADH (5′-ACC ATC GAG GAC ATA GAA-3′; forward primer, 5′-GTG GAG CCT GGG GTC AC-3′; reverse primer), and ALDH2 (5′-GCT GTC AGC AAG AAA ACA TTC CCC-3′; forward primer, 5′-CTT GTC AGC CCA GCC AGC ATA ATA-3′; reverse primer). The annealing temperature of 58°C and the cycle number of 45 were the parameters for the real-time PCR used to amplify these genes. The QuantStudioTM 3 (ABI) real-time PCR apparatus was utilized, and Macrogen (Seoul, Korea) produced the primers.
In triplicate trials, the data were calculated using mean values and standard deviations. To conduct statistical studies, SPSS 23 (SPSS Inc., Chicago, IL, USA) was used. The significance between controls and treatments was evaluated using the Duncan’s t-test, the Student t-test, or one-way analysis of variance. When p<0.05, differences were deemed significant.
Results
To investigate the effect of MC products on alcohol metabolism, male and female mice were orally administered MC products 30 min before alcohol administration. Blood samples were collected at 1, 3, and 5 h after alcohol administration to measure blood ethanol concentrations. In male mice, no statistically significant difference in blood ethanol concentration was observed between the MC product group and the alcohol-only group 1 h after alcohol administration. However, 3 h after alcohol administration, the blood ethanol concentrations in the Morning Care H, Morning Care PRESSON H, Morning Care PRESSON G, and Morning Care EX groups were 149, 103, 95, and 129% of the alcohol-only group (169%), respectively, showing a statistically significant decrease (p<0.05). Furthermore, 5 h after alcohol administration, the blood ethanol concentrations in the Morning Care H, Morning Care PRESSON H, Morning Care PRESSON G, and Morning Care EX groups were 90, 71, 60, and 53% of the alcohol-only group (113%), respectively, showing a statistically significant decrease (p<0.05). Similar results were observed in female mice. One hour after alcohol administration, there was no statistically significant difference in blood ethanol concentration between the MC product group and the alcohol-only group. However, 5 h after alcohol administration, the blood ethanol concentrations in the Morning Care H, Morning Care PRESSON H, Morning Care PRESSON G, and Morning Care EX groups were 89, 55, 51, and 65% of the alcohol-only group (111%), respectively, showing a statistically significant decrease (p<0.05) (Fig. 1A).
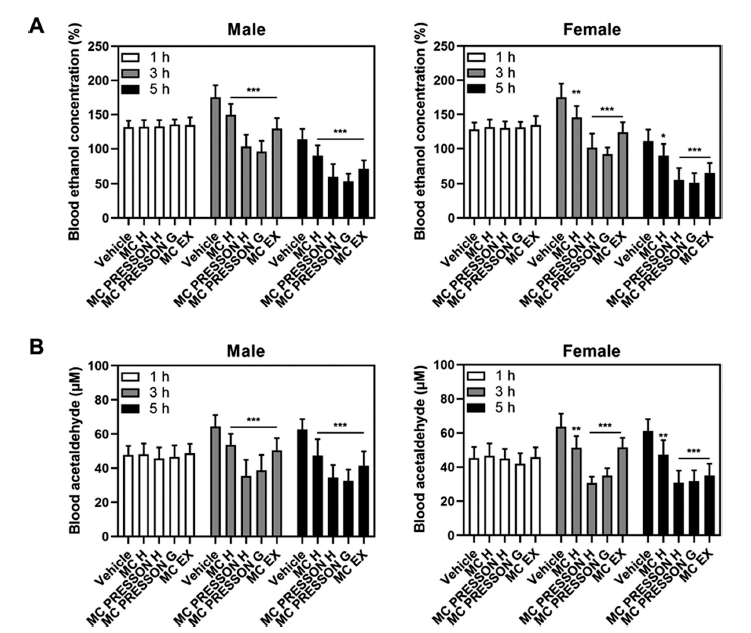
In male mice, blood acetaldehyde levels increased 3 h after alcohol administration and remained elevated at 5 h in the control group. The blood acetaldehyde concentrations at 3 and 5 h post-alcohol administration were significantly lower in the Morning Care H, Morning Care PRESSON H, Morning Care PRESSON G, and Morning Care EX groups compared to the control group (p<0.05). Notably, 5 h after alcohol administration, the blood acetaldehyde levels in the Morning Care PRESSON H and Morning Care PRESSON G groups were 34.5 μM and 32.5 μM, respectively, which is 0.54- and 0.51-fold lower than the control group at 62.7 μM. Similarly, in female mice, the blood acetaldehyde concentrations at 3 and 5 h post-alcohol administration were significantly lower in the Morning Care H, Morning Care PRESSON H, Morning Care PRESSON G, and Morning Care EX groups compared to the control group (p<0.05). Specifically, the Morning Care PRESSON G group showed a reduction to 0.47-fold and 0.50-fold compared to the control group at 3 and 5 h, respectively (Fig. 1B). These results indicate that Morning Care products reduce blood ethanol concentrations and the levels of acetaldehyde, a major cause of hangovers. This suggests that MC products are effective in relieving hangover symptoms.
We assessed ADH activity in the blood and liver of mice fed alcohol to determine whether MC products may prevent hangovers. The animals were both male and female. ADH activity was measured at 1, 3, and 5 h following alcohol feeding in mice that had received MC products 30 min prior. In male mice, ADH activity in the blood showed significant increases with the administration of MC products compared to the vehicle group. At 1 h, Morning Care PRESSON G exhibited significantly higher ADH activity compared to the vehicle group. This elevated activity persisted at 3 and 5 h, with the Morning Care PRESSON H group showing the highest activity compared to the vehicle (Fig. 2A).
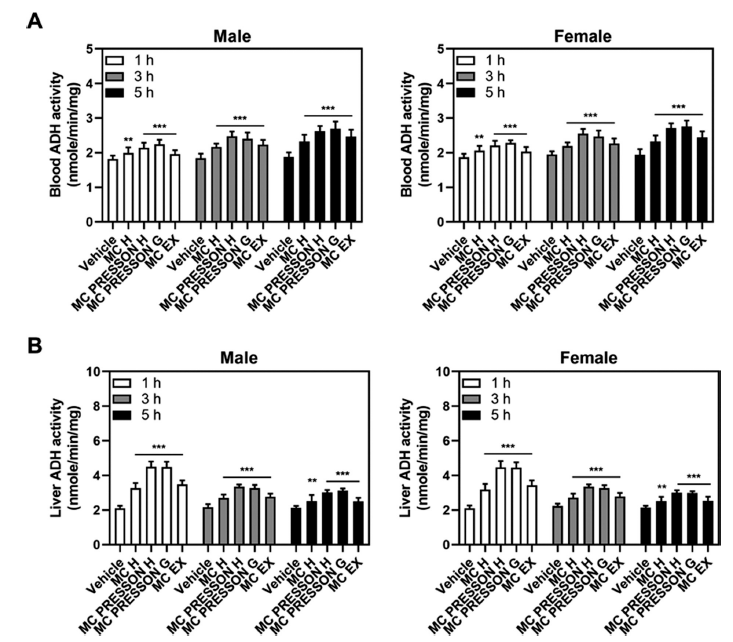
In the liver of male mice, ADH activity at 1 h was highest in the Morning Care PRESSON H group, indicating enhanced alcohol metabolism compared to the control group. While this elevated activity generally decreased by 3 h, it remained elevated compared to the control group, with the Morning Care PRESSON H group showing the highest ADH levels. By 5 h, ADH activity remained higher in all treatment groups compared to the control group, suggesting prolonged activation of alcohol metabolism (Fig. 2B).
For female mice, a similar result was observed in ADH activity. At 1 h, the Morning Care PRESSON G group exhibited the highest ADH activity in the blood, indicating a rapid metabolic response to alcohol. This elevated activity was sustained at 3 h, with the Morning Care PRESSON H group showing the highest activity. By 5 h, ADH activity remained significantly elevated across all treatment groups, suggesting a sustained metabolic effect (Fig. 2A).
In the liver of female mice, ADH activity was highest at 1 h in the Morning Care PRESSON H group, similarly to male mice. Although the activity generally reduced by 3 h, it remained increased compared to the control group, with the Morning Care PRESSON H group maintaining the highest ADH levels. By 5 h, ADH activity remained elevated in all treatment groups, indicating a prolonged enhancement in alcohol metabolism (Fig. 2B).
Next, we examined the ALDH activity in the blood and liver depending on the administration of MC products in alcohol-fed mice. The ALDH activity levels at 1 h in the blood of male mice were 2.32, 2.45, 2.47, and 2.31 nmole/min/mg in the Morning Care H, Morning Care PRESSON H, Morning Care PRESSON G, and Morning Care EX groups, respectively, compared to the control group (1.99 nmole/min/mg) showing a statistically significant increase (p<0.05). This elevated activity persisted at 3 and 5 h, with the Morning Care PRESSON G group showing the highest activity compared to the vehicle (Fig. 3A).
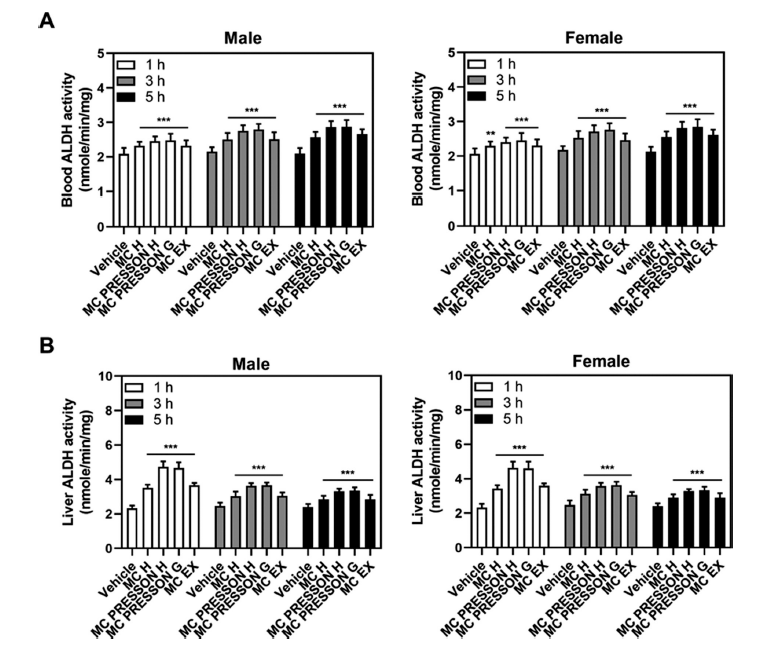
In the liver of male mice, ALDH activity in the MC product group showed overall higher levels than the control group at 1 h, especially peaking in the Morning Care PRESSON H group (4.73 nmole/min/mg). However, this elevated activity began to gradually decrease after 3 h but still remained higher compared to the control group (2.39 nmole/min/mg), particularly in the Morning Care PRESSON G (3.32 nmole/min/mg) and Morning Care PRESSON H (3.34 nmole/min/mg) groups (Fig. 3B).
For female mice, a similar trend was observed in ADH activity. Morning Care PRESSON G exhibited significantly higher ALDH activity compared to the vehicle group. This increased activity was sustained at 3 h. Additionally, 5 h after alcohol administration, ALDH activity in the blood of the Morning Care H, Morning Care PRESSON H, Morning Care PRESSON G, and Morning Care EX groups were 2.55, 2.81, 2.84, and 2.61 nmole/min/mg, respectively, compared to the alcohol-only group (2.13 nmole/min/mg), indicating a statistically significant increase (p<0.05) (Fig. 3A).
In the liver of female mice, ALDH activity was notably elevated at 1 h in the Morning Care PRESSON H group, similar to male mice. However, this increased activity began to decline gradually after 3 h yet remained higher compared to the control group, particularly in the Morning Care PRESSON G and Morning Care PRESSON H groups. Overall, the administration of MC products significantly increased ADH and ALDH activity in both blood and liver in male and female mice compared to the vehicle group (Fig. 3B). These results suggest that these products may effectively enhance alcohol metabolism, potentially mitigating the effects of alcohol consumption.
To address the expression level of the ADH and ALDH gene in mouse liver according to the administration of MC products to alcohol-fed mice, male and female mice were orally administered MC products 30 min before alcohol administration. Gene expression was determined at 1, 3, and 5 h after alcohol. In male mice, ADH gene expression did not show a statistically significant difference between the MC product group and the control group 1 h after alcohol ingestion, and although slightly higher at 3 and 5 h in the MC products treatment group, this difference was not statistically significant (Fig. 4A). When MC products were given to female mice prior to alcohol feeding, all groups’ levels of ADH and ALDH gene expression were similar after alcohol feeding. One, three, and five hours after alcohol feeding, there were no variations in the expression levels of the ADH and ALDH genes in any of the groups as compared to the control groups (Fig. 4B).
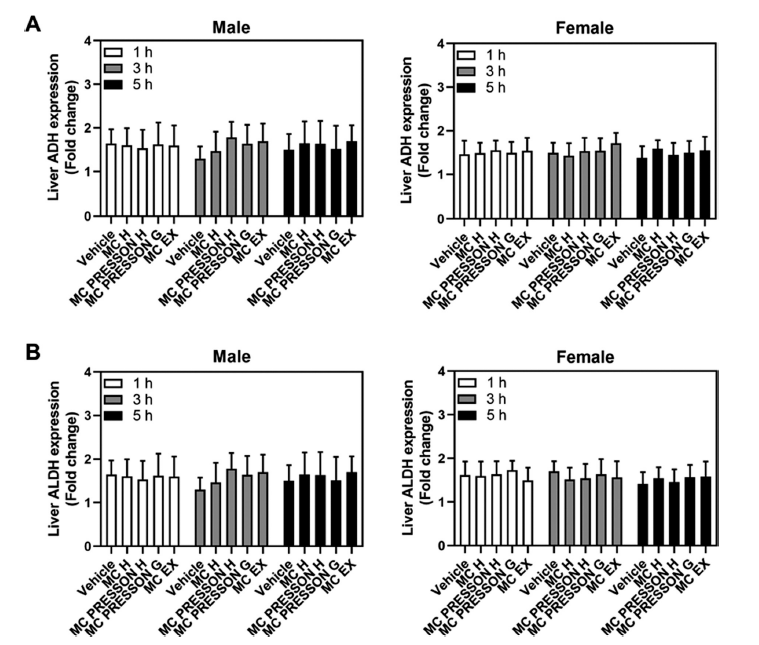
Discussion
The current study investigated the efficacy of MC products in alleviating hangover symptoms by focusing on their effects on alcohol metabolism in male and female mice. Due to differences in body size, body fat percentage, and alcohol metabolism rates, men typically metabolize about 20-30%, whereas women metabolize approximately 10% of the alcohol they consume (Frezza et al., 1990). Consequently, even with the same amount of alcohol consumed, reactions can vary between genders. Moreover, due to first-pass metabolism in the liver, where alcohol is metabolized before reaching systemic circulation, women tend to experience higher blood alcohol concentrations reaching the brain, leading to greater strain on the liver, quicker intoxication, and more pronounced hangover symptoms compared to men (Seyedsadjadi et al., 2022).
Recent research has shown that acetaldehyde removal is crucial for reducing alcohol-related hangovers. This suggests that the hangover may be impacted by increasing ADH and ALDH activity. According to Park et al., propolis reduces acetaldehyde in the liver and increases ADH and ALDH activity, which helps to alleviate hangovers (Lee and Park, 2021). Moreover, a plant-based extract mixture was found by Jung et al. to increase ADH and ALDH activity while lowering blood ethanol content (Jung et al., 2023). According to a study by Madoka et al., the peduncle of Hovenia dulcis and water extract of dried fruit enhanced ALDH and ADH activity (Niiya et al., 2024).
In this study, we directly compared the effectiveness of each product in relieving hangover symptoms and tested various products with different combinations of ingredients to evaluate which specific ingredients or combinations thereof were most beneficial for relieving hangovers. We observed that the administration of MC products before alcohol consumption led to significant alterations in alcohol metabolism in both male and female mice. Specifically, MC products effectively reduced blood ethanol concentrations at 3 and 5 h post-alcohol administration compared to the alcohol-only group, indicating enhanced metabolism of ethanol. This reduction in ethanol levels was accompanied by lower blood acetaldehyde concentrations, particularly notable at 5 h post-administration in both male and female mice. These findings suggest that MC products may facilitate the breakdown of ethanol to acetaldehyde and subsequently to acetate, thereby reducing the accumulation of acetaldehyde, a major contributor to hangover symptoms.
Moreover, our study demonstrated that MC products enhanced the activity of ADH and ALDH enzymes in the blood and liver of mice. ADH activity was notably increased in both male and female mice treated with MC products, particularly evident at 1 h post-alcohol ingestion. This increased enzyme activity persisted over time, indicating sustained enhancement of alcohol metabolism. Similarly, ALDH activity was significantly elevated in the MC product groups compared to the control groups, underscoring the products’ ability to promote the efficient conversion of acetaldehyde to acetate.
Interestingly, the expression levels of ADH and ALDH genes in the liver did not show significant differences between MC product-treated groups and control groups at 1, 3, and 5 h post-alcohol ingestion. This suggests that the observed increases in ADH and ALDH activities were likely due to post-translational modifications or enzyme activation rather than changes in gene expression.
Among the four MC products, Morning Care PRESSON H and Morning Care PRESSON G were the most effective in reducing blood ethanol and acetaldehyde concentrations and in enhancing ADH and ALDH activities, with Morning Care H and Morning Care EX also proving effective but to a slightly lesser extent. Morning Care PRESSON H stands out for its superior and sustained enzyme activation, particularly in both ethanol and acetaldehyde metabolism, while Morning Care PRESSON G is notable for its rapid efficacy in reducing ethanol levels and sustained high enzyme activity. In contrast, Morning Care H and Morning Care EX, though providing significant relief, were less effective compared to the PRESSON variants. Overall, the study suggests that Morning Care PRESSON H and Morning Care PRESSON G offer the most potent hangover relief among the tested products.
In conclusion, MC products enhanced alcohol metabolism by activating ADH and ALDH enzymes, thereby reducing hangover symptoms. Further research is needed to clarify the molecular mechanisms responsible for these effects and to evaluate their applicability. Our findings suggest that MC products could be beneficial in the effective management of hangovers, with significant implications for public health.