Introduction
Emulsifiers are often added during baking process and play an important role in dough stabilization, dough strengthening, crumb softening, and antistaling (Melis & Delcour, 2020). Commonly used baking emulsifiers include polysorbate 60, ethoxylated monoglycerides, succinylated monoglycerides, diacetyl tartaric acid esters of monoglycerides (DATEM), calcium stearoyl lactylate, sodium stearoyl lactylate, and propylene glycol alginate (PGA). The dough has a structure with gas cells and lipids dispersed in a gluten-starch matrix gas cell. Emulsifiers can adsorb on the surface of the gas cells to improve the stability of the gas cells, thereby stabilizing the dough, and can interact with the gluten to improve the gluten networking, thereby strengthening the dough (Mills et al., 2003;Melis & Delcour, 2020). Emulsifiers can also reduce starch retrogradation by inhibiting the recrystallization of amylose, thereby providing a dough softening effect that prevents bread from hardening after baking and cooling (Ahmad et al., 2014;Melis & Delcour, 2020).
In these days, there has been a growing interest in naturally occurring food additives due to consumer concerns (Asioli et al., 2017;Vargas & Simsek, 2021). Synthetic emulsifiers have been reported in an animal study to affect the gut microbiome, leading to inflammation and obesity (Chassaing et al., 2015). Therefore, there is a growing demand for natural emulsifiers in food industry. Currently known natural emulsifiers can be categorized into polysaccharides such as gums and pectins, proteins such as whey protein, casein, egg protein, soy protein, and gelatin, phospholipids such as lecithin, and small molecules including saponins (Ozturk & McClements, 2016).
Aqueous plant extracts from oats, beets, argan, canola, and chickpea have been reported to have emulsifying properties, primarily due to proteins and saponins (Ralla et al., 2017;Ralla et al., 2018;Lafarga et al., 2019). Polysaccharides, such as pectin and hemicellulose, may also be responsible for the emulsifying properties of plant extracts (Olorunsola et al., 2018). Polyphenols can exhibit Pickering emulsification effect by the formation of crystal structure and can enhance emulsifying properties through interaction with proteins (Zembyla et al., 2018;Zhang et al., 2023). It was also reported that a hot-water extract of chickpea, called aquafaba, has similar foaming properties to egg white and can partially replace eggs in cake making (Mustafa et al., 2018;Lafarga et al., 2019).
In this study, firstly, the hot-water extracts of pumpkin leaf, which is known to have strong antioxidant activity due to its high phenolic content (Kim et al., 2011), and of chickpea were prepared and investigated for their surface tension and foaming properties. Then, the effects of the plant water extracts on white pan bread baking were examined in comparison with the effect of propylene glycol alginate (PGA), a synthetic emulsifier widely used in bakery, to explore their potential as a natural emulsifier with antioxidant properties.
Materials and Methods
Pumpkin leaves and Canadian chickpeas were purchased from a local market (Hongcheon, Korea) and Daegu Agricultural Products Co., Ltd. (Gyeongju, Korea), respectively. Wheat flour, sugar, and salt, used in white pan bread baking, were purchased from CJ Co. (Seoul, Korea). Whole milk powder and unsalted butter were obtained from Seoul Dairy Cooperative (Seoul, Korea). Bakery yeast, dough enhancer, and propylene glycol alginate (PGA) were provided by SPC Research Institute of Food and Biotechnology (Seoul, Korea). Sodium carbonate (Na2CO3) was obtained from Daejung Co. Ltd. (Siheung, Korea). Folin-Ciocalteu’s reagent and gallic acid were purchased from Sigma-Aldrich (St. Louis, MO, USA). All other regents were of analytical grade.
Fresh pumpkin leaves were freeze-dried (FD-550, Tokyo Rikakikai Co., Ltd., Tokyo, Japan) and then ground at 25,000 rpm for 30s using a pulverizing machine (RT-N12HKW, Rong Tsong Precision Technology Co., Taichung, Taiwan), followed by sieving with a 300 mm sieve to prepare pumpkin leaf powder. The powder (25 g) was added to distilled water (475 g) at 80°C, stirred for 5 min, and extraction was performed in a shaking water bath at 80°C for 1 h. The powder suspension was then centrifuged (Supra R12, Hanil Scientific Inc., Gimpo, Korea) at 10000× g for 10m in, and the supernatant was collected to prepare hot-water extract of pumpkin leaf.
Fresh chickpeas were washed with tap water and then soaked in 3 times their weight in tap water at 25°C overnight. The chickpeas were removed and soaked in an equal weight of distilled water, then boiled for 12 min from the time the water started to boil with the lid closed and then left at room temperature for 5 h. The chickpea mixture was centrifuged at 10000× g for 10m in, and the supernatant was collected to prepare hot-water extract of chickpea.
The moisture, protein, lipid, carbohydrate, and ash contents of the plant water extracts were analyzed according to the methods of AOAC (AOAC, 2000). The moisture content was determined by mixing the plant water extracts with sea sand and drying it at 105°C for 24 h. The ash content was determined by carbonization of the extracts in an electric furnace (JFMP38, Jisico, Seoul, Korea) at 550°C overnight. The lipid and protein contents were determined by the Soxhlet and Kjeldahl methods, respectively, and the carbohydrate content was obtained by subtracting the amount of moisture, protein, lipid, and ash from the total amount of the extracts.
The total phenolic content of the plant water extracts was determined by Folin-Ciocalteu’s method with some modifications (Singleton & Rossi, 1965). Each plant water extract (200 mL) w as m ixed w ith 2 0 0mL Folin-Ciocalteu reagent in 2.6 mL distilled water and reacted for 6 min. Subsequently, 2 mL of 7% Na2CO3 was added and reacted in the dark at 25°C for 2 h. The absorbance was measured at 760nm using a UV spectrophotometer (UV-1800, Shimadzu, Tokyo, Japan). The total phenolic content was quantified using a standard curve with gallic acid as the standard, and expressed as mg gallic acid equivalent (GAE)/kg extract and mg GAE/g dry mass.
The surface tension (air-liquid interfacial tension) of the plant water extracts was measured by the Wilhelmy plate method at 25°C using a force tensiometer (K100, KRÜSS, Hamburg, Germany) (Phawaphuthanon et al., 2019). The solid content of the plant extracts was adjusted by dilution using citrate buffer (0.1 M, pH 5.6), and the PGA solution in citrate buffer was used as a control. Each plant water extract was placed in a sample vessel (SV20, KRÜSS) and the surface tension was measured using a platinum plate (PL01, KRÜSS). The surface tension was measured repeatedly at 2-min intervals until the standard deviation of the last 30 data was less than 0.1 mN/m.
The foaming properties of the plant water extracts were analyzed by measuring the foaming capacity and foam stability at 25°C according to the method of Kilicli and Toker (2022). A volume of 30 mL of each plant water extract was poured into a 500 mL beaker and whipped for 4 min using a hand mixer (5KHM5110, KitchenAid, St Joseph, MI, USA) to generate foam. The foaming capacity and foam stability were calculated according to the following equations (1) and (2), respectively.
The solid content of the plant water extracts was adjusted by dilution using citrate buffer (0.1 M, pH 5.6), and the PGA solution in citrate buffer was used as a control.
The white pan bread was prepared by the straight dough method according to AACC (2000) with some modifications, and its final composition is shown in Table 1. All ingredients except butter were mixed using a mixer (SP-800A, Spar Food Machine Co. Ltd., Taichung, Taiwan) at 130rpm for 3 min and 235 rpm for 2 min. The butter was then added and mixed at 130rpm for 3 min and 235 rpm for 10 min. The gluten window test was used to check for gluten formation, and if not enough gluten was produced, the dough was mixed for an additional 2 min at 235 rpm. The primary fermentation of the dough was performed at 27°C and 75% relative humidity (RH) for 1 h using a fermenter (Electric Proofer, PBC-PDM8, Ilsintech, Hanam, Korea), and then the dough was divided into 380g portions for preshaping. The intermediate proofing was carried out at 27°C, 75% RH for 30m in, after which the dough was molded. The final fermentation was performed at 38°C, 80% RH for 1 h, and if the bread did not rise sufficiently, the fermentation was continued for another 5 min until the bread filled at least 90% of the mold. The bread baking was conducted for 25 min in an oven (OV750, Living Well Korea, Seoul, Korea), preheated to a top temperature of 180°C and a bottom temperature of 220°C, followed by cooling for 1 h at room temperature.
A total of six types of white pan bread were prepared as summarized in Table 1. As controls, bread without PGA and plant water extracts (CPGA-) and bread containing only 0.05% (w/w) PGA (CPGA+) were prepared. Two types of bread containing pumpkin leaf water extract were prepared: one prepared with 0.05% (w/w) water extract (P0.05) and one prepared with 0.15% (w/w) water extract (P0.15), based on total solid content of the extract. Two type of bread containing chickpea water extract were also prepared: one prepared with 0.05% (w/w) water extract (A0.05) and one prepared with 0.15% (w/w) water extract (A0.15), based on total solid content of the extract.
The viscoelastic property of the dough was measured at 25°C using a hybrid rheometer (DHR-3, TA Instruments Inc., New Castle, DE, USA) according to McCann and Day (2013) with some modifications. A parallel-plate geometry with a diameter of 40m m was used, and the geometry gap was set to 2 mm. The dough was placed on the bottom of the rheometer, and the plate was slowly lowered to have a gap of 2.05 mm from the bottom. The excess dough that had spread outside the plate was removed, and the exposed surface was covered with Vaseline oil to prevent drying of the dough, after which the dough was allowed to rest for 10m in before analysis. The strain sweep test was performed in the range of 0.003-100% at a frequency of 1 Hz to determine linear viscoelastic region (LVR). The frequency sweep test was carried out in the range of 0.1-100 Hz at a strain of 0.1%, a value within the LVR.
The height, specific volume, and baking loss of the white pan bread prepared were measured. The height of the bread was measured based on the highest part. The volume of the bread was measured by the seed displacement method using sesame seeds, and the specific volume was calculated using equation (3) (Lee et al., 2017;Le Loan et al., 2021). The mass of the dough and the mass of bread after baking were measured, and the baking loss was calculated using equation (4).
The textural properties of the white pan bread prepared were analyzed by texture profile analysis (TPA) using a texture analyzer (TA1, AMETEK Lloyd Instruments Ltd., Largo, FL, USA) according to the AACC method (AACC, 2000) with some modifications. The crumb of the bread was taken from the middle of the bread, cut into cubes (2 cm × 2 cm × 2 cm), and then compressed in two cycles to 45% of the bread cube thickness using a cylindrical probe (50m m diameter) at a speed of 2 mm/s with a 10s waiting time between the compression. The hardness, springiness, chewiness, and cohesiveness of the crumb were determined using the TPA profiles obtained.
Results and Discussion
The proximate composition and total phenolic contents of hot-water extracts of pumpkin leaf and chickpea are shown in Table 2. No fat was detected in both hot-water extracts. For the pumpkin leaf water extract, the contents of protein, carbohydrate, and ash ranged from 0.4-0.6% (w/w), with a total solid content of only 1.5% (w/w), and the total phenolic content was measured to be 845.5 mg GAE/kg extract (56.8 mg GAE/g dry mass). The chickpea water extract had a relatively high protein and carbohydrate contents of 1.2% and 3.1%, respectively, and a total solid content of 5%, but a relatively low total phenolic content of 498.9 mg GAE/kg extract (10.0 mg GAE/g dry mass). The total phenolic and total solid contents of the chickpea water extract were similar to the reported values of aquafaba from commercial canned chickpeas (Raikos et al., 2020).
The surface tension of the two types of plant water extract and PGA solution was measured at 25°C with respect to total solid content (Fig. 1). The citrate buffer used to dilute the plant water extracts and prepare the PGA solution had a surface tension of 73.6 mM/m, similar to the surface tension of 25°C water of 72.0m N/m. The PGA solution (positive control) showed a surface tension of 44.7 mN/m at a PGA content of 2.0%, and the surface tension increased to 62.8 mN/m as the PGA content decreased to 0.001%. Huang et al. (2001) reported a similar surface tension for PGA solution (47.8 mN/ m at 0.5% PGA content).
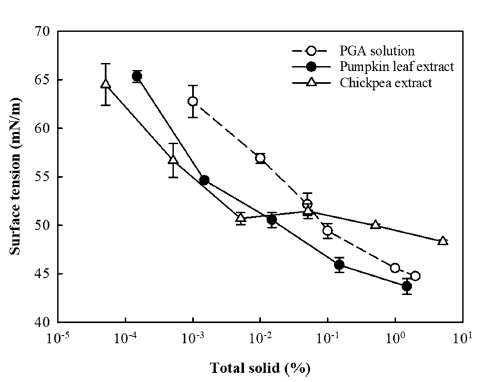
The surface tension of the pumpkin leaf water extract (1.5% total solid content) was 43.7 mN/m and also tended to increase with decreasing total solid content. At the same total solid content, the pumpkin leaf water extract showed a lower surface tension than the PGA solution (Fig. 1), indicating that the pumpkin leaf water extract may have better air-liquid interfacial activity, i.e., better foaming effect, compared to PGA. The surface-active properties of pumpkin leaf water extract are likely due to the combined action of several surface-active substances, including not only proteins (0.5%, w/w), but also polysaccharides such as pectin and hemicellulose, and saponins, which are expected to be present in trace amounts (Ralla et al., 2017;Olorunsola et al., 2018;Ralla et al., 2018). In addition, phenolic compounds in pumpkin leaf water extract (845.5 mg GAE/kg extract) can form crystals, exhibiting the Pickering effect, or interact covalently or non-covalently with proteins to enhance the emulsifying effect of proteins (Zembyla et al., 2018; Zhang et al., 2023).
The chickpea water extract (5.0% total solid content) exhibited a surface tension of 48.3 mN/m, which is slightly higher than the pumpkin leaf water extract (1.5% total solid content) and PGA solution (2.0%) (Fig. 1). This indicates that despite its higher total solid content, the chickpea water extract has a relatively low air-liquid interface activity compared to the pumpkin leaf water extract and PGA solution. However, for the chickpea water extract, unlike the other two types of liquids, there was no significant change in surface tension when the total solid content was reduced to 0.005% (Fig. 1). When the total solid content was further reduced below 0.005%, the surface tension increased to a level comparable to that of pumpkin leaf water extract. The surface activity of chickpea water extract is mainly due to the proteins present in large quantities (Mustafa et al., 2018;Lafarga et al., 2019).
The foaming capacity and foam stability of the two types of plant water extract and PGA solution were determined at 25°C as a function of total solid content (Fig. 2). For the PGA solution (positive control), the surface tension increased as the PGA content decreased from 2.0% to 0.001% (Fig. 1), but the foaming capacity was measured in the range of 213-290% over a PGA content of 0.01-1.0% and showed no dependence on PGA content (Fig. 2a). This is probably because as the PGA content decreased, the surface tension of the solution decreased, but the solution viscosity also decreased, which facilitated the entry of air for foam formation (Nilsen-Nygaard et al., 2016;Phawaphuthanon et al., 2019). The foam stability exhibited similar values of 51-55% in the PGA content range of 0.1-1.0%, but decreased sharply as the PGA content was lowered, showing a value of 21% when the PGA content reached 0.01% (Fig. 2b). The results indicated that when the PGA content was lower than 0.1%, the foaming capacity was maintained due to the lower viscosity, but the stability of foams was greatly reduced.
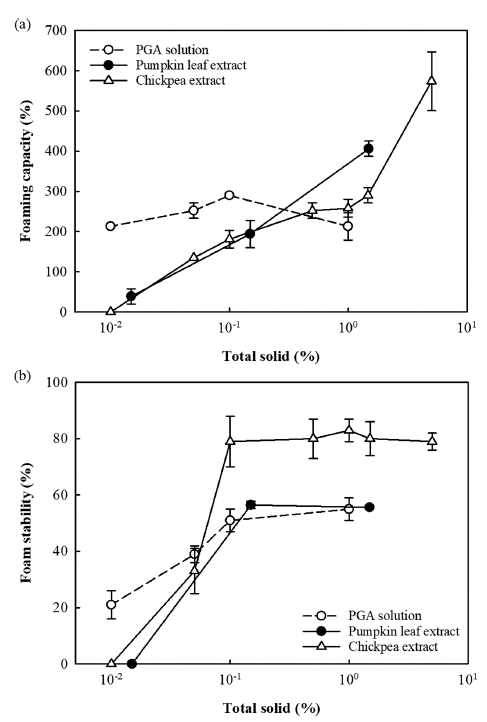
For the pumpkin leaf water extract (1.5% total solid content), the foaming capacity was 406%, which is about twice as high as the 1.0% PGA solution (Fig. 2a). However, as the total solid content decreased, the foaming capacity decreased sharply, resulting in a lower foaming capacity compared to the PGA solution, and almost no foam was formed when the total solid content reached 0.01%. The decrease in foaming capacity with decreasing total solid content may be due to the increase in surface tension (Fig. 1). The foam stability of the pumpkin leaf water extract was about 56% in the range of 0.15-1.5% total solid content, which was similar to the values of PGA solution with 0.1-1.0% PGA content, but it decreased sharply as the total solid content decreased, showing 0% when the total solid content reached 0.01% (Fig. 2b). The results indicated that the pumpkin leaf water extract (1.5% total solid content) had forming properties comparable to the PGA solution immediately after extraction, but relatively poor foaming properties when diluted.
The chickpea water extract (5.1% total solid content) showed a relatively high foaming capacity of 574%, compared to the pumpkin leaf water extract (1.5% total solid content) and PGA solution (1.0%), despite its high surface tension (Fig. 2a). This is probably because proteins, the main surface-active substance in the chickpea water extract, were adsorbed at the air-liquid interface, forming a protein film (Phawaphuthanon et al., 2019). However, the foaming capacity decreased rapidly and was lower than that of the pumpkin leaf water extract, as the total solid content decreased to 1.5% (Fig. 2a). The foaming capacity steadily decreased as the total solid content was further reduced, with no foam formation observed at a total solid content of 0.01% (Fig. 2a). The foam stability of the chickpea water extract was significantly better than that of the pumpkin leaf water extract or PGA solution when diluted until the total solid content reached 0.1% (Fig. 2b), due to the protein film formed at the air-liquid interface (Phawaphuthanon et al., 2019). However, when the total solid content was lower than 0.1%, the foam stability decreased sharply and showed values similar to those of the pumpkin leaf water extract (Fig. 2b).
The viscoelastic properties of white pan bread dough containing either 0.05% or 0.15% (based on total solid content) of the two plant water extracts or PGA are shown in Fig. 3. In the strain sweep test (at 1 Hz frequency), all doughs, including the control, exhibited similar G' (storage modulus) and G'' (loss modulus) values and showed a characteristic of solid with G' greater than G'' over the strain range tested (Fig. 3a). The LVR was observed in the strain range of 0.01-0.12%, and above this strain range, a nonlinear region was observed where the moduli decreased with increasing strain due to deformation of the dough structure. In the frequency sweep test (at 0.1% strain), all doughs also exhibited similar G' and G'' values, with both moduli increasing with increasing oscillatory frequency (Fig. 3b). The tan d (=G''/G') decreased with increasing frequency for frequencies below 1 rad/s, but tended to increase with increasing frequency for frequencies above 1 rad/s, with values in the range of 0.1-0.5 for almost all frequencies tested (Fig. 3c). When frequency <1 rad/s, increasing the frequency can cause a kneading effect, which strengthens the gluten structure and improves the elasticity, resulting in a decrease in tan d (Correa et al., 2010;Ziobro et al., 2013). However, when frequency > 1 rad/s, the increase in frequency may break down the gluten structure formed, reducing the elasticity, and thus increasing tan d (Correa et al., 2010;Ziobro et al., 2013). Considering that G'>G'' in all measurements (meaning that elasticity prevails over viscous nature), the dependence of the moduli on the oscillatory frequency, and the range of tan d values, the prepared dough can be classified as a weak gel (Ziobro et al., 2013).
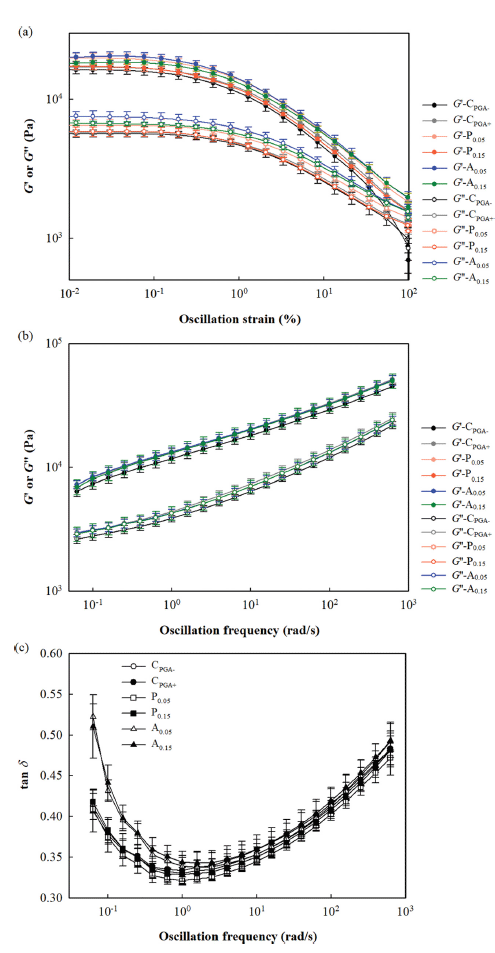
The white pan breads baked in this study did not show any significant difference in appearance, regardless of whether PGA or plant water extracts were added (Fig. 4). The height, specific volume, and baking loss of the white pan bread prepared with either 0.05% or 0.15% (based on total solid content) of the two plant water extracts or PGA were determined (Table 3). For the height, the bread with 0.15% pumpkin leaf water extract (P0.15) had the highest value of 13.9 cm, while the bread with 0.05% chickpea water extract had the lowest value.
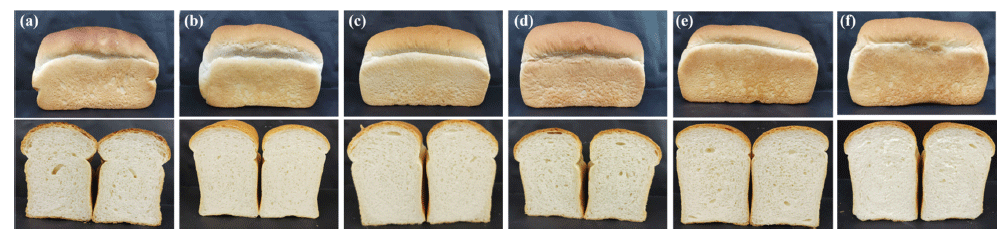
For the specific volume, the negative control bread (CPGA-) and the two breads prepared with 0.05% plant water extracts (P0.05 and A0.05) showed similar values (4.94-4.97 cm3/g), while the breads prepared with 0.05% PGA or 0.15% plant water extracts had larger values (5.09-5.24 cm3/g). The increase in the specific volume indicates that the gas cells formed during the baking were better developed and stabilized, which is probably due to the adsorption of PGA or the plant water extracts at the gas cell interface and the resulting surface-active behavior (Melis & Delcour, 2020).
For the baking loss, the negative control bread (CPGA-) showed the highest value (15.3%), while the other breads had lower values of 12.0-13.0%. The addition of PGA or the two types of plant water extract may have increased the waterholding capacity of the dough, thereby reducing the baking loss (Kotoki & Deka. 2010;Chauhan et al., 2016).
It should be noted here that polyphenols can positively or negatively affect yeast metabolism and gluten formation, depending on the type of polyphenols (Mekoue et al., 2019;Xu et al., 2019;Kalinina et al., 2023). Therefore, polyphenols in the plant water extracts may also be one of the reasons why the extracts have positive effects on the baking properties, but further investigation is needed to clarify the role of polyphenols.
The textural properties of the crumb of white pan bread baked with either 0.05% or 0.15% (based on total solid content) of the two plant water extracts or PGA are shown in Fig. 5. The negative control bread (CPGA-) showed the highest crumb hardness of 0.57 N (Fig. 5a). This is because CPGA- has a small specific volume due to low gas cell formation capacity and a high baking loss due to low water-holding capacity (Table 3), resulting in the formation of a relatively hard bread crumb. The crumb hardness was significantly reduced with the addition of plant water extracts or PGA, especially with 0.15% pumpkin leaf water extract (P0.15) or 0.05% PGA (CPGA+), which resulted in a low hardness of 0.38 N (Fig. 5a). This is because the plant water extracts and PGA adsorbed on the gas cell interface of the dough to stabilize the gas cells, and interacted with gluten to enhance gluten networking to strengthen the dough, thereby increasing the specific volume and improving the water-holding capacity to reduce baking loss, resulting in the formation of a larger and softer loaf (Mills et al., 2003;Kotoki & Deka. 2010;Chauhan et al., 2016;Melis & Delcour, 2020).
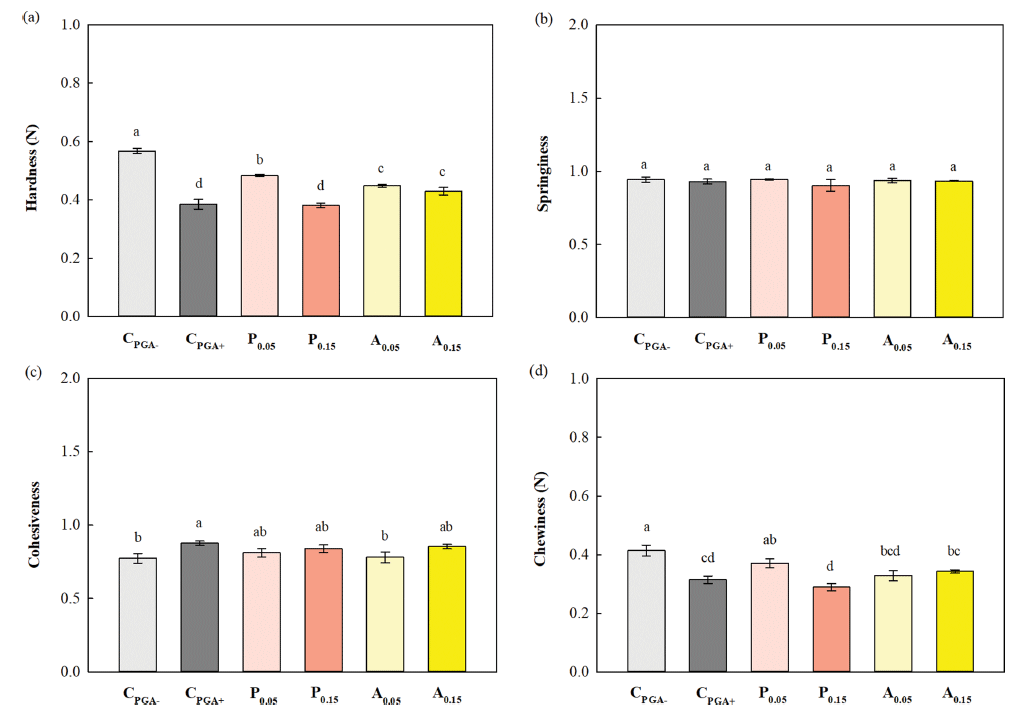
No significant differences were observed between the bread samples in springiness, which represents the elasticity of bread texture, and cohesiveness, which indicates the cohesive property of the texture (Fig. 4b and 4c). Thus, the chewiness (= hardness × springiness × cohesiveness), which represents the chewability of bread, showed a similar trend to the hardness, with CPGA- bread having the highest chewiness (0.41 N), and P0.15 and CPGA+ breads having significantly lower values (0.28- 0.31 N) (Fig. 5d).
Conclusions
When total solid content ³0.15%, the hot-water extract of pumpkin leaf showed better air-liquid surface tension compared to the hot-water extract of chickpea or aqueous PGA solution, and similarly good foaming capacity with slightly lower foam stability than the chickpea water extract. The plant water extracts did not significantly affect the viscoelasticity of bread dough having a weak gel structure, and when added at 0.15%, the bread specific volume increased and the baking loss was reduced. In particular, when 0.15% pumpkin leaf water extract was added, the crumb hardness and chewiness decreased to the level of the crumb prepared with 0.05% PGA. This is because the surface-active substances in pumpkin leaf water extract adsorbed to the surface of gas cells in the dough, increasing the stability of gas cells, and also interacted with proteins to strengthen the gluten structure, taking over the role of a bakery emulsifier, resulting in the formation of a larger and softer bread. The results indicate that the pumpkin leaf water extract can be used in a baking process as an effective natural emulsifier with high-phenolic content (56.8 mg GAE/g dry mass). Further research is needed to determine the effects of adding pumpkin leaf water extract, even in trace amounts, on bread flavor.