Introduction
Extrusion cooking is presently the principal processing technology used for texturization of meat analog. It can create the meat-like cell structure from soy protein employing high moisture, temperature, and shear and eliminate the bitter taste and odor (Samard et al., 2019). Extrusion process using mechanical technology is possible to the “bottom-up” approach as directly apply for the produce of meat-like structure via the self-assembling into fibrils (Sun et al., 2020). There are also industrial advantages in terms of cost effectiveness and capability for producing large mount in shot time (Harper, 1979).
Moreover, extrusion using high moisture content (40-80%) could produce highly fibrous meat analog comparable to meat, poultry, or fish muscle (Lin et al., 2002). Furthermore, extrusion cooking is influenced by extrusion type (low or high moisture). High moisture extrusion typically controlled above 50% moisture content with heating that uses twin screw equipped with cooling die. It prevents rapid expansion and aligns the protein mass into the fibrous structure using laminar flow (Maung, 2020). The mechanism has an effect on producing a more meat-like texture of extruded meat analog than low moisture extrusion (Samard et al., 2019).
Soy materials are the most commonly used in meat analog because of their characteristics functional properties including water holding, gelling, fat-absorbing, and emulsifying capacities. There are several types of base sources in the meat analog market such as defatted soy flour (DSF), isolated soy protein (ISP), soy protein concentrate (SPC) and full-fat soy, etc. (Kyriakopoulou et al., 2019).
The primary protein source is ISP containing over 90% of protein, miner moisture content, and ash, which is currently used in high-moisture extrusion (Ma & Ryu, 2019). On the other hand, full-fat soy (FFS) contains about 22% fat as well as 40% protein, 33% carbohydrate, minor fiber as nutritional values (Akingbala et al., 1995). It has weak off-flavor, good color, and appearance (Neson & Leigh, 1983). Moreover, FFS is so cheap and easy to get flour comparing to ISP that is high-cost and required with several steps. But FFS commonly has been used to enhance the nutrition and addition of gluten protein making a dough due to the lower protein content compared with other soy products (Singh et al., 2007). However, Maung (2020) reported that FFS-based extruded meat analog had the higher textural properties compared with ISP-based extruded meat analog. Therefore, FFS-based could be suitable alternative of ISP with purified conventional soy base.
In addition, the additives of extruded meat analog commonly influence flavor, texture, and nutrition values of final products (Liu et al., 2005). Over a long time, mushrooms have been a high-quality food sources containing bioactive nutrients that can good acts for the meat analog (Arora et al., 2017). These can be used in well balanced diets that possess low fat and high contents of dietary fiber, and carbohydrate, valuable salts, vitamins, polyphenolic compounds, and amino acids (El-Refai et al., 2014). Also, mushrooms possess dietary fiber which can increase levels of physical properties such as texture, gelling, thickening, emulsification, and stabilization (Pakhare et al., 2018;Quan et al. 2019).
Oyster mushroom (Pleurotus ostreatus) especially is one of the most common cultivated type which has excellent flavor and taste. Pleurotus species among mushrooms have strong and chewy texture responsible for dense structure in fungal tissue of fruit body (Yeob et al., 2016). Moreover, the polyphenol extract in vegetables such as oyster mushroom and protein interaction, which enhance cross-linked network in formation of extruded meat analog as well as free radical scavenging activity (Ma & Ryu, 2019;Quan et al., 2019).
Previous studies on extruded meat analog have typically been performed by using ISP-based types (Ma & Ryu, 2019;Samard et al., 2019;Cho & Ryu, 2020) and DSF (Cho & Ryu, 2017; Liu et al., 2005), and SPC (Chiang et al., 2020). However, the study on full fat soy (FFS)-based extruded meat analog has been few found. Therefore, this study was investigated effects of oyster mushroom addition (0, 4, 8, 12%) on physicochemical properties of FFS-based extruded meat analog.
Materials and Method
Full fat-soy (FFS) was obtained from Qone Co. Ltd. (Incheon, Korea), wheat gluten (WG), and corn starch (CS) were purchased from Roquette Freres (Lestrem, France), and Qone Co. Ltd. (Incheon, Korea), respectively. Oyster mushroom (Pleurotus ostreatus) powder was purchased from Ingreen (Pocheon, Korea).
The materials of FFS-based meat analog were composed of 50% full-fat soy (FFS), 40% wheat gluten (WG), 10% corn starch (CS). These base mixtures including FFS+WG+CS (w/ w) were added at the ratio of 100:0, 96:4, 92:8, 88:12 (base mixture: oyster mushroom, w/w) as FFS-based mixture and the protein contents of four mixtures were 73.25%, 69.00%, 57.99%, and 53.52% (dry basis), respectively. The protein contents were determined by ninhydrin method (Starcher, 2001).
The mixtures were passed through between the sieves of 60 mesh (Screen US Standard Sieve Series) and maintained in plastic zipper bags at room temperature before extrusion process. All chemical reagents and standards of analysis for the study were of analytical grade.
Extrusion processes were conducted by using a co-rotating intermeshing twin-screw extruder (THK31T, Incheon Machinery Co., Ltd., Inchen, Korea). The screw configuration with a screw diameter of 3 cm and a length of 69 cm (L:D, 23:1) and the extruder equipped with cooling die (long-slit die formed) with the diameter of 7 × 1 × 50 cm (W × H × L) are shown in Fig. 1(a) & (b).
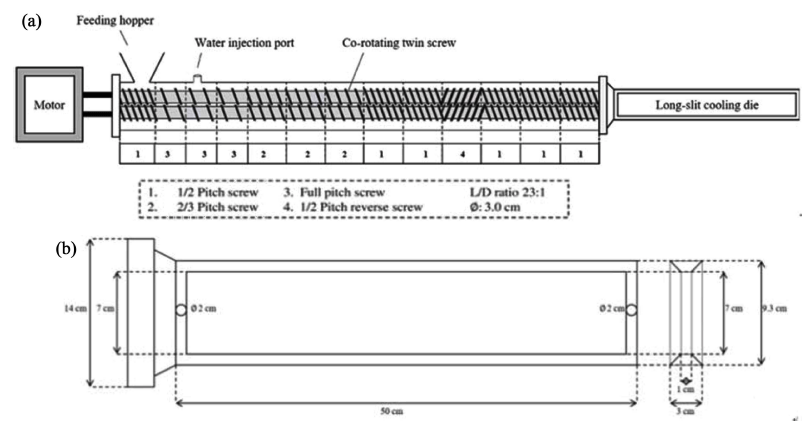
Extrusion conditions for texturization of FFS-based meat analog were fixed the screw speed of 150 rpm, moisture content of 55%, feed rate of raw materials at 100 g/min, barrel temperatures of 100, 170, and 130°C from feeding to die zones. The long-slit die equipped with running cooling water at 20-25°C from temperature controller (Duksan Cotran Co., Ltd. Daegu, Korea) was used in high moisture extrusion cooking. The water for the feed moisture was controlled by injecting it into extruder through constant flow pump. The samples of extruded meat analog were directly stored in refrigerator (FR-S690FXB, Klasse Auto Co., Ltd., Seoul, Korea) at -20°C until conducting analysis. The physical properties were determined using the cutting samples of 1.5 × 1.0 × 1.5 cm (W × H × D), and the samples of freezedried, ground and sieved by passing through between 70 mesh (212 μm screen Standard Sieve Series) and 50 mesh (300-μm screen Standard Sieve Series) were used for analysis of chemical properties.
Texture profile analysis (TPA) was determined with Sun rheometer (Compac-100 II, Sun Sci. Co., Tokyo, Japan) equipped with 10 kg load cell and a cylinder probe of 25 mm in diameter. Each sample of 1.5 × 1.0 × 1.5 cm (W × H × D) was measured with vernier caliper (CD-15CPX, Mitutoyo Co., Ltd., Kawasaki, Japan). The hardness, cohesiveness, springiness, and chewiness were measured according to the analysis method by Maung (2020). As measurement conditions, the samples were compressed to 75% if its original height (10 mm) at a cross-head speed of 60 mm/ min with the distance between two supports of 13 mm, the angle of probe type of 65° and maximum peak stress of 10 kg. Determinations were based on ten measurements and then were calculated as described by Breene (1975). For cutting strength, a knife probe equipped with 2 kg load and 100 mm/min were measured by Cho & Ryu (2020) using 6 × 2 × 1.5 cm (W × H × L). The results were averaged from ten measurements.
The integrity index was determined by the method of Maung (2020). The sample (5 g of dry basis) was immersed in a conical flask containing 100 mL of distilled water and then soaked at 80°C. After 30 min, the sample was placed in PAC- 60 autoclave (Lab House Co., Ltd., Seoul, Korea) at 121°C for 30 min and transferred again into 100 mL distilled water to cool and was homogenized by homogenizer (T10 Basic Ultra- Turrax, IKA Co., Ltd., Staufen, Germany) for 1 min at 14,450 rpm. The sample was directly drained through the sieve of 20 mesh and dried at 105°C for 4 h. The integrity index in percent was calculated as the residue weight divided by dried sample weight.
Water holding capacity (WHC) was determined by method of Lin et al. (2002). Approximately 25 g of samples (dry basis) were weighed and rehydrated at 50°C in the distilled water for 12 h followed by draining for 5 min. The water holding capacity was calculated by subtracting dry sample weight from wet sample weight divided by the original sample weight.
Nitrogen solubility index (NSI) was determined by the method of Cho & Ryu (2020). The freeze-dried and ground sample (1.5 g) was put into 75 mL of KOH solution and was stirred in shaker (SI-300R, Jeiotech Co., Ltd., Seoul, Korea) at 200 rpm. Solution (50 mL) was centrifuged at 3000 rpm for 30 min to acquire 0.5 mL of supernatant. Soluble nitrogen content was measured by using ninhydrin method (Starcher, 2001). The total nitrogen content was measured by completely hydrolyzing 1.5 g of the sample in 6 N HCl for 24 h at 100°C. The hydrolysates were dissolved in 75 mL of distilled water. The 0.5 mL of the supernatant was collected, and it was measured by using ninhydrin method (Starcher, 2001). NSI was determined with the following equation:
The total phenolic content (TPC) was determined by the method of Thin et al. (2016). The sample (approximately 1 g) was extracted with 10 mL phosphate buffered saline solution (PBS) for 2 h at room temperature. Then, the extracted sample was centrifuged at 3,000 rpm for 30 min. The 0.3 mL of the supernatant was taken from extraction and was mixed with 1.5 m L of 10% (v/v) in Folin-Ciocalteu Reagent. The solution was stirred and reacted for 5 min. The 1.5 m L of Na2CO3 solution (60 g/L) was added to this solution and then placed in incubation at room temperature for 2 h. The solution of sample was read at 765 nm using spectrophotometer (Biowave 3, Biochrom WPA, Co., Ltd., Sanghai, China). The concentration of TPC was measured by using an equation obtained from the gallic acid standard curve.
The DPPH (2,2-diphenyl-1-picrylhydrazyl) radical scavenging activity was determined according to the method of Brand- Williams et al. (1995). The extraction of sample (approximately 1 g) was done with 80% methanol at room temperature for 2 h and centrifuged at 3,000 rpm for 30 min. The DPPH solution was prepared by adding 0.0024 g of DPPH reagent to 100 mL of methanol, and the solution (3.9 mL) was added to 0.1 mL of supernatant. After incubation at room temperature for 30 min in the dark, the absorbance was read at 515 nm using spectrophotometer (Biowave 3, Biochrom WPA, Co., Ltd., Sanghai, China). The DPPH radical scavenging percentage was determined with the following equation:
where Abscontrol is the absorbance of the control, and Abssample is the absorbance of the sample.
All experiments were performed in triplicate. Analysis of variance (ANOVA) and mean values were compared by using the Duncan’s multiple range tests at 95% of significance. All the statistical analysis was conducted using the SPSS for Window 12.0 software (SPSS, Chcago, IL, USA).
Result and Discussion
Crude protein contents of the raw mixture of FFS-based extruded meat analog with oyster mushroom contents are presented in Table 1.
Crude proteins of raw mixture decreased with the increase in oyster mushroom contents from 0 to 12% (73.25, 69.00, 57.99, and 53.52%). This result was because oyster mushroom has composition of the majority of carbohydrates, ash, fiber (about 65%), and about 34% of crude protein as lower level than that of a base mixture (FFS+WG+CS). Therefore, the addition of oyster mushroom attributed to decreasing the protein digestibility, nitrogen solubility index after extrusion cooking (Maray et al., 2018).
Texture profile properties including hardness, springiness, cohesiveness, chewiness, and cutting strength of FFS-based extruded meat analog with different mushroom contents are summarized in Table 2.
![]() |
The highest hardness, and chewiness were observed as 18.93 N, 12.15 N in FFS-based extruded meat analog with 12% mushroom contents. The hardness, chewiness increased with the increase in oyster mushroom contents. Cutting strength including transversal and longitudinal section force, which also increased with the increase in oyster mushroom contents from 0 to 12% (625.93, 681.00, 710.07, and 762.80 g/cm2). These might be due to protein-polyphenol conjugates which resulted in complex effects of cross-linked formation in meat analog (Quan et al., 2019). A similar result was found by Ma & Ryu (2019) who revealed that hardness and chewiness of ISP-based extruded meat analog increased with the increase in green tea contents 0, 5, 10, and 15% whereas springiness and cohesiveness indicated inconstant patterns like the present study (Table 2). In contrast, Cho & Ryu (2020) reported that all the hardness, cohesiveness, springiness, chewiness, and cutting strength of ISP-based extruded meat analog which consistently increased with the increase in oyster mushroom contents (0, 4, 8, and 12%) These may be related in the report that cohesiveness, and springiness of extruded meat analog was mainly influenced by cross-linking formation about the internal resistance of structure (Ma & Ryu, 2019). Especially, springiness mainly is dependent on recovery between first and second compression in extruded meat analog. Moreover, these factors commonly were affected by protein content that related to disulfide bonding (Samard et al., 2019). Therefore, FFSbased containing lower protein and higher fat content showed the inconstant patterns compared with ISP-based (Cho & Ryu, 2020).
On the other hand, according to the study by Cho & Ryu (2017), the textural properties including cutting strength as well as hardness, springiness, cohesiveness, and chewiness of DSF-based extruded meat analog which decreased with the increase in tuna sawdust contents as animal additive. Similar finding was reported by Chiang et al. (2020) who also stated that SPC-based extruded meat analog with 0% beef bone hydrolysate obtained the highest hardness and chewiness.
Based on the results, the addition of plant materials containing polyphenol compound, fiber, and polysaccharide, which could lead to rheological stability responsible for composite matrix in structure compared to the addition of animal sources (Jia et al., 2015). In addition, the oyster mushroom and green tea classified in plant additives possess polyphenol compounds which lead to gelling, insoluble, crosslink with protein interaction (Ma & Ryu, 2019;Quan et al., 2019).
The digital photographs for confirming the visible structure of FFS-based extruded meat analog with different oyster mushroom contents are exhibited in Fig. 2. The compact layers with fibrous structures revealed with the increase in oyster mushroom contents from 0% to 12% (Fig 2a-d). This result was in accordance with the finding of Cho & Ryu (2020) who reported that the layers and fiber arrangements in ISP-based extruded meat analog increased with the increase in oyster mushroom contents (0, 4, 8, and 12%). Based on the results, the addition of oyster mushroom contributed to creating the fibrous structure in extruded meat analog, which may be due to protein-polyphenol complex related to cross-linking as well as disulfide bonding (Samard et al., 2019;Quan et al., 2019).
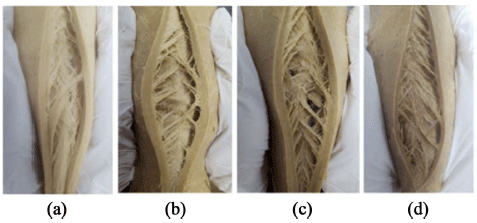
The integrity index refers to the residue texture after meat analogs is hydrated, autoclaved, homogenized, and dried, which is an important property that indicates the quality of meat analogs (Samard et al., 2019;Ma & Ryu, 2019). Integrity index of FFS-based extruded meat analog with different oyster mushroom content is presented in Fig. 3(a). The highest integrity index was indicated as 81.75% at 12% oyster mushroom contents. Integrity index increased with the increase in oyster mushroom contents. This result was in accordance with the finding of Cho & Ryu (2020) who reported that the integrity index of ISP-based extruded meat analog also increased with the increase in oyster mushroom contents with the same patterns. Similar result was found by Ma & Ryu (2019) who reported that the integrity index of ISPbased extruded meat analog increased with the increase in green tea contents. The increase in integrity index may be due to combination of polyphenol extract in oyster mushroom and soy protein which led to improving the texturization responsible for protein-polyphenol complex during extrusion cooking (Ma & Ryu, 2019). Moreover, the higher integrity index may be due to improving the rheological stability with polysaccharide and fiber interaction in formation during the pressure and high temperature via extrusion cooking.
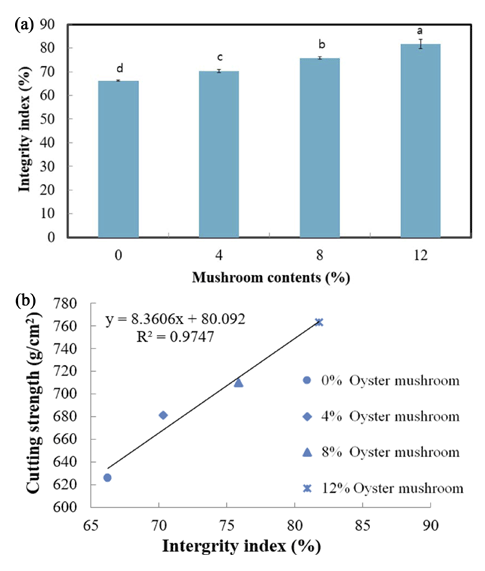
In addition, the relationship between integrity index and cutting strength of FFS-based extruded meat analog is shown in Fig. 3(b). Integrity index and cutting strength were positively correlated (R2 = 0.9747). Similar study was found by Samard et al. (2019) who stated that higher cutting strength led to higher integrity index related to harder and more texturized formation.
Nitrogen solubility index (NSI) was used to determine the water dispersible nitrogen referring to the soluble protein, and protein denaturation that was generated during the manufacture of meat analog (Samard et al., 2019). NSI of FFS-based extruded meat analog with different oyster mushroom contents is presented in Fig. 4(a). The highest NSI was observed as 66.42% in FFS-based extruded meat analog with 0% oyster mushroom content while the lowest was obtained as 47.49% at 12% oyster mushroom contents. NSI decreased with the increase in oyster mushroom contents. These results were in accordance with the findings of Cho & Ryu (2020) who reported that the addition of oyster mushroom led to decreasing the NSI. The researcher also stated that the decrease in NSI was due to the increase insoluble structure responsible for promoting the polyphenol with protein matrix by increasing the oyster mushroom (Ma & Ryu, 2019).
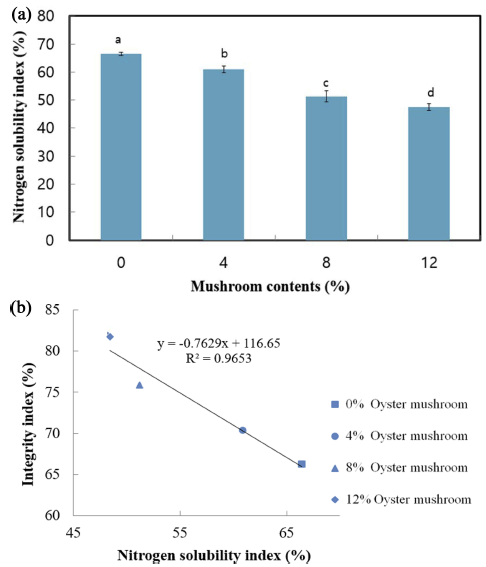
Furthermore, the relationship between NSI and integrity index of FFS-based extruded meat analog presented in Fig. 4(b). NSI and integrity index of FFS-based extruded meat analog were negatively correlated (R2 = 0.9653). Likewise, similar findings were found by Cho & Ryu (2020) who expressed that NSI of ISP-based extruded meat analog decreased with the increase in oyster mushroom contents due to less solubility responsible for producing cross-linked structure.
Water holding capacity (WHC) is defined as the ability that lead to the amount of water in shrunken structure of meat samples without changing cell membrane after rehydration (Lin et al., 2002).
WHC of FFS-based extruded meat analog with different oyster mushroom contents is shown in Fig. 5. The highest WHC was obtained as 330.59% in FFS-based extruded meat analog with 4% oyster mushroom contents while the lowest was indicated as 289.08% at 12% oyster mushroom contents. The addition of oyster mushroom led the patterns like the results of cohesiveness and springiness in this study.
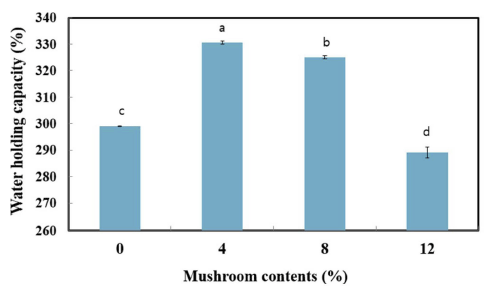
In contrast, Cho & Ryu (2020) reported that WHC of ISP based extruded meat analog consistently decreased with the increase in oyster mushroom contents. Likewise, previous studies of extruded meat analog using soy protein materials such as ISP, DSF which commonly can find the inverse correlation between WHC and texture properties (Cho & Ryu, 2017;Ma et al., 2018, Samard et al., 2019). Therefore, WHC also commonly affected by porous structure related to air cell size in extruded meat analog. However, in the present study, WHC increased at 4% oyster mushroom contents while it decreased at 8, 12% as inconstant patterns (Fig. 5). These results suggested that the oyster mushroom content of over 8% led to increasing strong polyphenol-protein conjugate related to decrease the air cell’s relaxation in structure, which contributed to the decrease WHC (Ma & Ryu, 2019).
Total phenolic content as botanical antioxidants possessed in oyster mushroom is essential to physiological human health that can be conflict free radical substance, which has played an important role in inhibiting aging processes such as cancer, and various inflammatory disease as well as functional decline (Khatun et al., 2015). TPC of FFS-based extruded meat analog is shown in Fig. 6. In the present study, the highest TPC was observed as 52.09 mg GAE/g in FFS-based extruded meat analog with 12% oyster mushroom contents whereas the lowest was obtained as 28.54 mg GAE/g at 0% oyster mushroom contents. TPC increased with the increase in oyster mushroom contents. A similar finding was also found by Ma & Ryu (2019) who revealed that the addition of green tea in ISP-based extruded meat analog led to increasing the TPC. Moreover, Maung (2020) also similarly reported that TPC and flavonoid content of FFS-based was higher than in ISP-based extruded meat analog.
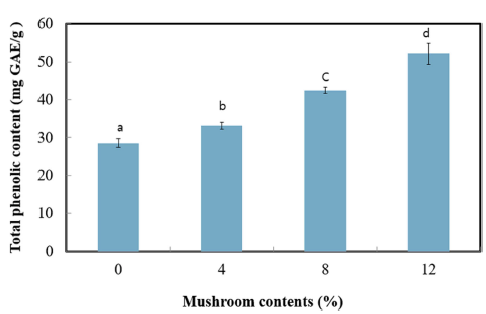
Based on the results and reports, these exhibited that FFS typically has a lot of isoflavones classified in total phenolic compounds compared with ISP which was almost removed during the purifying process. Therefore, FFS-based revealed the higher TPC than ISP-based extruded meat analog in TPC.
In addition, TPC in FFS-based extruded meat analog increased with the increase in oyster mushroom contents. Rahimah et al. (2019) stated that the phenolic components in oyster mushroom which possesses typical types including vanillic acid, myricetin, caffeic acid, tannic acid, chrysin. Overall, the addition of oyster mushroom containing phenolic compounds that contributed to the increase TPC in extruded meat analogs, which also attributed to polyphenol-protein conjugates related to the antioxidant activity (Khatun et al., 2015).
Determination of DPPH radical scavenging activity which reflects the antioxidant content in foods that is important for the protection of deleterious changes and loss of commercial and nutritional values (Kedare & Singh, 2011).
DPPH radical scavenging activity of FFS-based extruded meat analog with different oyster mushroom contents is exhibited in Fig. 7(a). In the present study, the highest DPPH radical scavenging activity was observed as 11.79% in FFSbased extruded meat analog with 12% oyster mushroom contents while the lowest was revealed as 6.54% at 0% oyster mushroom content. the DPPH radical scavenging activity of FFS-based extruded meat analog increased with the increase in oyster mushroom contents 0, 4, 8, and especially at 12%. This result indicated that the addition of oyster mushroom led to polyphenol-protein conjugates which contributed to the higher antioxidant activity (Quan et al., 2019). Similar trends were found by Cho & Ryu (2020) who revealed that DPPH radical scavenging activity of ISP-based extruded meat analog increased with the increase in oyster mushroom contents.
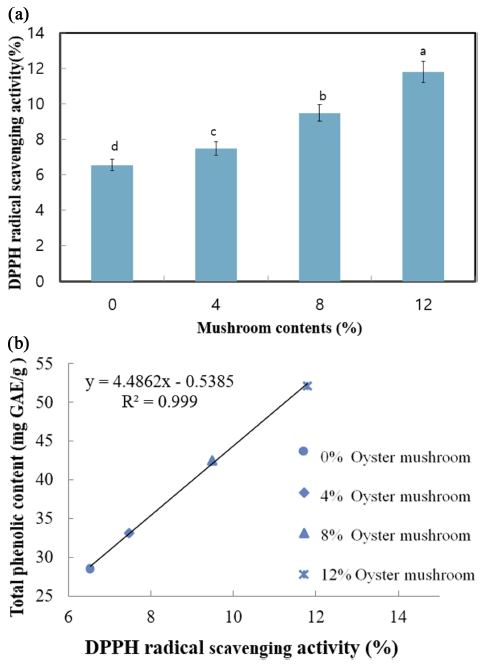
Moreover, Maung (2020) who stated that FFS-based had the higher antioxidant properties than in ISP-based extruded meat analog due to the various phenolic compounds in full-fat soy meal. The higher DPPH radical scavenging activity in FFS without purifying process may be due to rich α-tocopherol and sterol contents as well as unsaturated fatty acid, and selenium (Mattaus & Qzcan, 2014;Kaur et al., 2018). The α-tocopherol classified in vitamin E commonly contributes to the protection of sensitive fatty acids that are influenced by free radicals (Rani et al., 2007). Similar finding was also reported by El- Demerdash (2004) who revealed that α-tocopherol in vitamin E and selenium could be beneficial in alleviating aluminum toxicity. Therefore, the increase in FFS-based extruded meat analog may be due to FFS containing α-tocopherol or selenium which can cause the decrease in levels of free radicals (Finke, 2002).
In addition, the relationship between TPC and DPPH radical scavenging activity of FFS-based extruded meat analog is displayed Fig. 7(b). TPC and DPPH radical scavenging activity of FFS-based extruded meat analog were positively correlated (R2 = 0.9990).
Previous studies have been reported that the phenolic components in oyster mushroom attribute to antioxidant activity (Khatun et al., 2015;Yeob et al., 2016;Um et al., 2010). Cooney & Ross (1987) also stated that the vanillin classified in plant had an effect on decreasing the nitrite levels by inhibiting the N-nitration related to radicals. Similar study was found by Khatun et al. (2015) who showed that chelating ability in oyster mushroom was positively correlated with total phenol content.
Based on the above studies, the antioxidant property of extruded meat analog incorporating plant materials were mainly influenced by total phenolic substances.
Conclusion
In this study, the addition of oyster mushroom enhanced textural properties including hardness, chewiness, cutting strength, integrity index as well as antioxidant activity while nitrogen solubility decreased. These suggested that the addition of oyster mushroom in FFS-based extruded meat analog lead to polyphenol-protein conjugate, which contributed to cross-linking complex. In addition, cohesiveness, springiness, and water holding capacity showed the inconstant patterns. This may be caused from low protein and high fat content in FFS-based. Also, WHC had the inconstant patterns with the increase in oyster mushroom contents. This revealed that the oyster mushroom content of over 8% led to increasing strong polyphenol-protein conjugate which contributed to the decrease WHC. The decrease in NSI was due to lower dispersion and break of formation with the increase in oyster mushroom contents. As the increasing oyster mushroom contents, the TPC and DPPH radical scavenging activity increased due to several polyphenol substances and ability of free radical scavenging in oyster mushroom.
Based on the present results, FFS-based extruded meat analog was comparable to ISP-based extruded meat analog. Moreover, the addition of oyster mushroom improved the textual properties and antioxidant activity of FFS-based extruded meat analog that can be good acts as an additive of extruded meat analog.