Introduction
Flavonoids are polyphenol secondary metabolites found in plants. They can be found in organs of plants. These plant organs include leaves, fruits, roots, and stems (Karak, 2019). They comprise a big part of polyphenolic compounds with basic γ-benzopyrone structure and hydroxylated phenolic substances. They are synthesized by the phenyl-propanoid pathway in plants, which occurs in reaction to microbial infection (Dixon et al., 1983;Kelly et al., 2002). Flavonoids are well known as phytochemicals, which are chemicals from plants that have diverse biological functions as well as being essential nutrients (Gharras, 2009). They have many beneficial biochemical effects and antioxidant benefits in relation to various illnesses, including cancer, Alzheimer’s disease atherosclerosis. (Burak & Imen, 1999;Panche et al., 2016). Flavonoids have a broad spectrum of biological activities and health-promoting effects as antioxidants, anti-inflammatories, antimicrobials, and anti-carcinogens. These properties along with their ability to regulate major cellular enzyme functions make flavonoids an essential component of various nutraceutical, pharmaceutical, medicinal, and cosmetic applications. The activities of flavonoids depend on the structure. Also, their chemical nature is dependent upon their structural class, level of hydroxylation, substitutions and conjugations, and level of polymerization (Kelly et al., 2002). Functional hydroxyl groups in flavonoids influence their antioxidative activities by scavenging free radicals as well as chelating metal ions in biological systems. Moreover, flavonoids are thought to be profound inhibitors of some enzymes, such as xanthine oxidase (XO) and cyclo-oxygenase (COX), which are associated with hyperuricemia and inflammation in biological systems, respectively (Umamaheswari et al., 2011;Madeswaran et al., 2012). It has been suggested by many studies that flavonoids protect against many infectious diseases as well as degenerative problems and agingassociated illnesses including cardiovascular diseases and cancer (Cook & Samman, 1996;Pandey, 2007;Murlidhar et al., 2010;Kumar et al., 2013).
Some have suggested to utilize flavonoids in the development of food therapies and new food preservatives because of their antimicrobial properties. Flavonoids can be widely found in plant-derived foods and beverages, such as fruits, teas, cocoa, and wine. In food products, they are usually labeled as dietary flavonoids and are categorized into subgroups, including flavones, flavonols, flavanone, flavanol, anthocyanins, and isoflavones (Panche et al., 2016;Karak, 2019). It has been known that these flavonoid subgroups are synthesized in certain parts of different plants. As a result of this, plant-derived foods and beverages get their unique and various organoleptic characteristics. There are approximately 6,000 flavonoids that contribute to the color, aroma, taste and other organoleptic characteristics of plant-based foods. Because they are known to have health benefits and help to prevent chronic degenerative diseases, they are often referred to as “functional ingredients” or “health-promoting biomolecules” (Nijveldt et al., 2001;Karak 2019). The goal of this review is to navigate the potential valuable biological properties of flavonoids according to their chemical structures and to describe flavonoid-rich food sources.
Chemical Structures and Classification of Flavonoids in Food Plants
Flavonoids are a class of low molecular weight substances that have variable phenolic structures. They can be found in various plants. Initially, flavonoids were believed to be a kind of vitamin, termed vitamin P, until they were discovered to be in fact flavonoids. Flavonoids’ basic chemical structure is the flavan nucleus arranged in a diphenylpropane skeleton. They are labeled as A, B, and C (Fig. 1). Flavonoids are frequently found in glycosylated or esterified forms, which are based on 2-phenyl-chromone nucleus (Fig. 2). To date, there are known to be over 6000 varieties of flavonoids. (Middleton 1998;Panche et al., 2016;Wang et al., 2018). Chemically, flavonoids have a fifteen-carbon skeleton with a diphenyl propanoid C6- C3-C6 backbone (Fig. 2) that combines two benzene rings connected by a heterocyclic pyran ring with three carbons (Fig. 2) (Terahara, 2015). Flavonoids are often hydroxylated at positions 3, 5, 7, 3', 4', and 5'. Generally, they exist in the form of glycosides. When glycosides are structured, the usual location of the glycosidic linkage is 3 or 7 of the basic flavonoid structure. The carbohydrate can be rhamnose, glucose, glucorhamnose, galactose, or arabinose (Middleton, 1984).
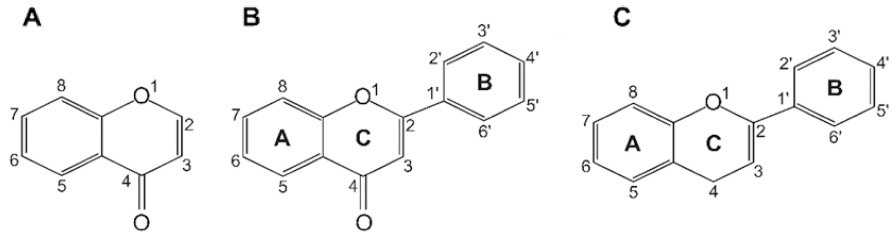
Flavonoids can be categorized into different subgroups, including flavones (1), flavonols (2), flavanones (3), flavanols (4), isoflavones, (5) and anthocyanins (6); the general chemical structures of the backbones of each of these subgroups are shown in Table 1. The subgroup into which each flavonoid is categorized depends on its degree of oxidation (how oxidized it is), the substitution pattern of its C ring (what the pattern of substitution of its C ring is), and B ring’s position of connection (and where the connection position of B ring is) (Fig. 2). Various individual flavonoid compounds within each subgroup differ in terms of the substitution pattern of A and B rings (Middleton, 1998). When the B ring is linked at position 2 of the C ring, these subgroups are flavones, flavonols, flavanones, flavanols, and anthocyanins. On the other hand, when the B ring is linked at position 3 of the C ring, they are isoflavones (Table 1). Flavones and flavonols represent flavonoids belonging to the 2-benzo-γ-pyrone category and they contain the most compounds. Flavanone and flavanols contain saturated C2=C3 bonds while coexisting with certain flavones and flavonols found in plants (Wang et al., 2018). Flavonols differ from flavanones in that they have a hydroxyl group at position 3 and a C2=C3 double bond (Narayana et al., 2001). Anthocyanins are a collection of significant chromene pigments that produce the distinct colors of plants and exist in the structure of ions (Wang et al., 2018). Below, I discuss each of the flavonoid subgroups in greater detail.
![]() |
Flavones are a subgroup of flavonoids. They have a double bond between position 2 and 3 and a ketone at position 4 of the C ring, as shown in Table 1. Flavones cannot be found as commonly as flavonols in fruits and vegetables even though they widely exist in various plant-based foods, chiefly in the form of glycosides of luteolin and apigenin (Gharrs, 2009). In most flavones in fruits and vegetables, hydroxyl groups are at position 5 of the A ring and hydroxylation at others, e.g. at position 7 of the A ring or positions 3' and 4' of the B ring. Though, this may differ depending on the taxonomic classification. Parsley and celery are known to be the two main dietary sources of flavones (Table 2). However, citrus fruit peels contain large amounts of polymethoxylated flavones tangeretin, nobiletin, and sinensetin, which are the most hydrophobic flavonoids (Nielsen et al., 2003;Manach et al., 2004).
Classification | Flavonoids | Dietary Sources | References |
---|---|---|---|
Flavone | Apigenin | Celery, parsley seed, chamomile | Sung et al. (2016) |
Luteolin | Parsley, broccoli | Justesen & Knuthsen. (2001) | |
|
|||
Flavonol | Quercetin | Vegetables, kale, onions, berries | Justesen & Knuthsen. (2001), Manach et al. (2004) |
Kaempferol | Leeks, spinach, broccoli | Manach et al. (2004), Dabeek & Marra. (2019) | |
Rutin | Citrus fruits, buckwheat, apples, cherries, red peppers | Atanassova & Bagdassarian. (2009), Kreft et al. (1999) | |
|
|||
Flavanone | Naringenin | Citrus fruits, grapefruits | Ho et al. (2000), Vallverdú-Queralt et al. (2012) |
Hesperetin | Citrus fruits, oranges | Manach et al. (2004), Mohammadi et al. (2019) | |
Eriodictyol | Citrus fruits, lemons | Manach et al. (2004), Miyake et al. (1997) | |
|
|||
Flavanol | Catechin | Green tea, apricots, red wine | Wang et al. (2000), Gharras (2009) |
Epicatechin | Cacao, black tea | Arts et al. (2000), Manach et al. (2004) | |
|
|||
Isoflavone | Daidzein | Soybeans | Reinli & Block. (1996), Laurenz et al. (2017) |
Genistein | Soybeans | Raurenz et al. (2017) | |
|
|||
Anthocyanin | Pelargonidin | Blackcurrants | Slimestad & Solheim. (2002) |
Cyanidin | Blackberries | Ding et al. (2006) | |
Delphinidin | Black grapes | Farida et al. (2016) | |
Pheonidin | Blueberries | Manach et al. (2004) | |
Malvidin | Strawberries | López de Las Hazas et al. (2017) |
Flavonols are the most common flavonoids and can be found in a variety of foods. They are known as the building blocks of proanthocyanidins (Panche et al., 2016). As shown in Table 1, flavonols, unlike flavones, have a hydroxyl group at position 3 of the C ring, in which, glycosylation may also occur. The associated sugar moieties in the glycosylated form are very often glucose and rhamnose. However, they may be other forms of sugars as well. (Neo et al., 2008). Given their hydroxylation and glycosylation patterns, flavonols may be the most commonly found flavonoids subgroup in fruits and vegetables. Flavonols found in foods can mainly be characterized by kaempferol and quercetin (Manach et al., 2004;Gharras, 2009), which are structurally optimized for free radical scavenging and therefore exhibit strong antioxidant activity. The best sources of flavonols include onions, kale, leeks, broccoli, cherry, apple, and berries (Table 2).
Flavanones are an important subgroup of flavonoids that are present only in citrus fruits. Flavanones are also called dihydroflavones. Unlike flavones, they have a saturated C ring, therefore, a double bond in the C ring exist between positions 2 and 3. This is the only chemical structural difference between flavones and flavanones (Iwashina, 2013). Flavones are generally glycosylated by disaccharides at position 7 and form either neohesperidose or rutinose. Neohesperidose gives a bitter taste, while rutinose is flavorless. The non-sugar components that result from the hydrolysis of glycoside flavanones are aglycones. The main aglycones in citrus fruits, are hesperetin, naringenin, and eriodictyol that can be found in oranges, grapefruits and lemons, respectively (Table 2). These compounds impart the bitter taste in citrus fruits. Also, they have pharmacological significance due to their antioxidative, anti-inflammatory, and cholesterol-lowering activities in biological systems (Iwashina, 2013;Terahara, 2015).
Flavanols are also known as catechins. They are the 3- hydroxy derivatives of flavanones, as shown in Table 1. Another name for flavanols is flavan-3-ols (e.g., catechins, epicatechins, gallocatechins) due to the hydroxyl group bound to position 3 of the C ring. Flavanols exist both as monomers or catechins and polymers or proanthocyanidins. Catechins are found in many foods, such as apricots, red wines, green tea, and chocolate (Table 2). However, green tea is the richest source of them all. There is up to 200 mg catechins is an infusion of green tea, while black tea made from green tea leaves contain fewer monomer flavanols like catechins. This is because monomer flavanols are oxidized during processing and become complex condensed polyphenols like proanthocyanidins. Proanthocyanidins are referred as theaflavins and thearubigins, which are dimers and polymers, respectively (Day et al., 2001). Proanthocyanidins are also known as condensed tannins. They are chemically dimers, oligomers, and polymers of catechins. They are connected together by links between C4 and C8 (or C6) (Souquet et al., 1996). The main flavanols that can be found in fruits are catechins and epicatechins. Moreover, leguminous plants, grapes and tea are a rich source of gallocatechins and epigallocatechins (Bravo, 1998;Manach et al., 2004).
A large and very unique subgroup of flavonoids are isoflavones. They are provided in the human diet primarily by soybeans and their derived products (Matthies et al., 2008). Isoflavones can exist as aglycones or glycosides, and can solely be found in leguminous plants. Isoflavones have a typical chemical structure, with the B ring of the flavone molecule attached to carbon 3 of the heterocycle, unlike other subgroups of flavonoids (Bravo, 1998). Daidzin (daidzein glycoside), genistin (genistein glycoside), and glycitin (glycitein glycoside) are known as the soy-derived isoflavones (Table 2). Isoflavones are well known to be phytoestrogens, based on the estrogenic activity, shown in animal models. They also show great potential in fighting several diseases in biological systems (Szkudelska & Nogowski, 2007). It was also discovered that isoflavones work as precursors in developing phytoalexins that are produced by plants as a reaction to pathogens (Aoki et al., 2000;Dixon & Ferreira, 2002).
Anthocyanins are flavonoids synthesized via the phenylpropanoid pathway. Anthocyanins are also a significant group of pigments that are water-soluble. They produce colors, blue, purple, and red, of certain flowers and fruits. The term “anthocyanin” means glycosides of anthocyanidins, such as pelargonidin, cyanidin, delphinidin, peonidin, and malvidin (Mazza & Francis, 1995;Bravo, 1998;Prior & Wu, 2006). They can be found mainly in fruits and their outer cell layers. Such fruits include blackcurrants, blackberries, black grapes, blueberries, and strawberries (Table 2). Mostly, anthocyanins are glycosylated at the C3 position. Besides C3, sugars may be connected at any one of the hydroxyls at C5, C7, C3′, C5′, and even C4′ (Mazza & Miniati, 1993;Takeoka & Dao, 2002;Anna, 2005). The anthocyanin compounds are glycosylated polyhydroxy and polymethoxy derivatives of 2-phenylbenzopyrylium (flavylium) salts. The most common sugars are glucose, galactose, rhamnose, and arabinose (Wu & Prior, 2005;Asif, 2015). The acylation of sugar moiety may be occurred by aromatic acids—mostly hydroxycinnamic acids such as caffeic, ferulic, р-coumaric, or sinapic acids. The number of compounds may increase greatly, by the number of possible combinations of positions of sugars and acyl moieties and positions and numbers of hydroxyl and methoxy groups on the anthocyanidin skeleton. (Wu & Prior, 2005;Mateus et al., 2006). The pH and methylation or acylation at the hydroxyl groups on the A and B rings are responsible for the color of anthocyanin (Iwashina, 2013).
Biological Properties of Flavonoids
Naturally-derived antioxidants are dietary components. They can decrease the damage to the tissue done by free radicals and reactive oxygen species (ROS) (Asif, 2015). Both enzymatic and non-enzymatic defense systems exist in most living organisms. These defense systems work against excessive production of free radicals and ROS. However, many exogenous elements, e.g. diet, smoking, use of alcohol and drugs and age, negatively affect these defense systems. As a result, the redox equilibrium that is formed in healthy conditions is disturbed (Leutner et al., 2001;Tapas et al., 2008). ROS-containing free radicals and endogenous antioxidants must maintain this homeostatic balance in order to maintain healthy tissues. In many oxidative illnesses caused by tissue injury, large amounts of free radicals and ROS could be found. Injuries caused by free radicals can be protected by flavonoids in variety of ways. Broadly speaking, free radical scavenging is the fundamental main mechanism of their antioxidant quality. The reducing activities of their phenolic hydroxyl groups enable flavonoids to donate hydrogen (Verma et al., 2012;Wang et al., 2018). In most cases, the position and number of hydroxyl groups in flavonoids are linked to their antioxidant activity. Ring B hydroxyl groups in flavonoids donate hydrogens to hydroxyl, peroxyl, and peroxynitrite radicals, and form strong flavonoid radicals. It is suggested that in order to have antioxidant capacity, there should be more than two hydroxyl groups in ring B. This can be proved by the significant increase of antioxidant effects in flavonoids that have such a structure. (Celik & Kosar, 2012). According to Hanasaki et al. (1994), some flavonoids scavenge precise superoxides, while other flavonoids search for the highly reactive oxygen-derived radical peroxynitrite. Flavonoids that have two hydroxyl groups in ring B, including epicatechin and rutin, are powerful scavengers of highly reactive oxygen-derived radicals. Vaya et al. (2003) note that two hydroxyl groups in the B ring of flavonoids acts as strong inhibitors on lowdensity lipoprotein oxidation in vitro. As suggested in a recent study by Federica et al. (2018), fruits like mahaleb cherry and blackcurrant, which contain non-acylated anthocyanins, exhibit much higher antioxidant capacity due to their scavenging of ROS radicals than black carrots and black tomatoes, which contain acylated anthocyanins with hydroxycinnamic acid derivatives. The potential antioxidative effect of anthocyanins appears to be dependent on the differences in their chemical structures. These structures are differentiated by the number and position of hydroxyl groups and conjugation groups, the level of glycosylation, and the presence of donor electrons in the ring structure along with acylation by aromatic acids (Giusti & Wrolstad, 2003;Reis et al., 2016). Therefore, the onset and worsening of autoimmune diseases, cardiovascular diseases, neurovascular diseases and other oxidative illnesses may be able be prevented with the help of antioxidants that scavenge free radicals and ROS. Natural plant-based antioxidants, primarily flavonoid phenolic compounds, may be more effective antioxidants than regular vitamins, such as vitamin C, vitamin E, and β-carotene. Although, these vitamins can also neutralize free radicals generated by carcinogenicity and cardiovascular and neurovascular changes brought on by aging (Yu, 1994;Tapas et al., 2008). Flavonoids are most found in fruits and vegetables thus, including fruits and vegetables in a regular diet may help protect humans from oxidative damage as they block and destroy free radicals and ROS.
Lipid peroxidation is generally caused by oxidative stress. Naturally occurring flavonoids can help reduce oxidative damage by different mechanisms (Kumar et al., 2013). Free metal ions strengthen the forming of ROS by reducing hydrogen peroxide and generating a highly reactive hydroxyl radical (·OH). This hydroxyl radical is a powerful oxidant produced in biological systems through the Fenton reaction (Fe2+ + H2O2 → Fe3+ + ·OH + OH-) (Wang et al., 2018;Collin, 2019). Flavonoids can chelate the transition metals that promote hydroxy radical formation (Wang et al., 2018). Trace metals connect at certain positions of the individual rings of flavonoids, as shown in Fig. 3. Particularly, quercetin is known for its iron-chelating and iron-stabilizing features (Van Acker et al., 1996a). Anthocyanins like cyanidin, with their 3', 4'- dihydroxy groups, have also been said to prevent lipid peroxidation brought about by copper metal by quickly chelating metal ions to establish strong anthocyanin–metal complexes (Reis et al., 2016). According to Kong et al. (2003), on the activity of anthocyanins against lipid peroxidation catalyzed by a high concentration of copper (80 μM), delphinidin was the best inhibitor of lipid peroxidation, followed by cyanidin and malvidin. The number of hydroxyl groups in ring B seems to make the biggest difference in inhibition of lipid peroxidation induced by a high level of metal ions in anthocyanins. Additionally, it has been reported that catechols with hydroxyl groups at the 3 and 4 positions of the B ring are effective inhibitors of lipid peroxidation. They scavenge ROS radicals such as peroxyl, superoxide, and peroxynitrite (Kelly et al., 2002). Epicatechin and rutin, which contain a catechol system, are also reported to effectively scavenge radicals and block lipid peroxidation in vitro (Kerry & Abbey, 1997). The 3'4' catechol structure in flavonoids’ B ring is a prominent feature of the most powerful scavengers of peroxyl, superoxide, and peroxynitrite radicals. For example, luteolin is a far stronger scavenger of peroxyl radical, compared to kaempferol. Even though both are identical in hydroxyl configurations, B ring catechol is missing in kaempferol (Van Acker et al., 1996b). The peroxynitrite scavenging ability of catechin attributes to its B ring catechol (Kerry & Rice-Evans, 1999;Heijnen et al., 2001). Oxidation of flavonoids happens on the B ring when the catechol is present. As a result, a strong ortho-semiquinone radical is formed through electron delocalization. Flavones without catechol or o-trihydroxyl (pyrogallol) systems result in fairly unstable radicals that may be less effective scavengers (Mora et al., 1990;Burda & Oleszek, 2001;Sekher Pannala et al., 2001).
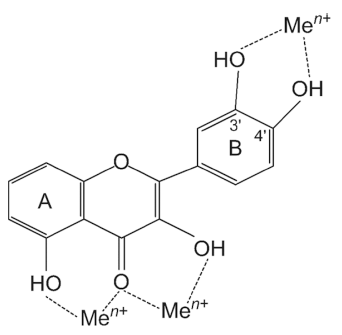
Body tissues have a biological response to harmful stimulants, which is referred to as inflammation. This response occurs to protect the body from harmful stimuli, including pathogens, cell damage, tissue injury, and chemical irritants. It involves immune cells, blood vessels, and chemical mediators. Inflammation begins when immune cells migrate from blood vessels and chemical mediators are released at the tissue damage site (Ferrero-Miliani et al., 2007;Karak, 2019). COX is an endogenous enzyme that acts as a catalyst when arachidonic acid is converted into prostaglandins (Smith et al., 2000). There are two isoforms of enzymes: COX-1 and COX- 2. The former is a constitutive enzyme and produces prostaglandins, whereas the latter synthesizes prostaglandins that brings about inflammation and pain (Kurumbail et al., 1996). Flavonoids have been found to have anti-inflammatory affects in many studies. A current study performed using in silico methods explored the idea that some flavonols and flavones with a double bond at the 2 and 3 positions could be referential inhibitors of COX-2 (D’Mello et al., 2011). Evaluation of flavonoids such as hesperitin, genistein, and daidzein containing a C2=C3 double bond was done to see their inhibitory activity of COX in a study by Madeswaran et al. (2012). The docking results revealed that some select flavonoids that have a C2=C3 double bond within their structures, such as rutin, a flavonol, and farobin-A, a flavone, exhibited increased aldose reductase inhibitory activity due to their structural parameters, which may be closely related to the treatment of diabetes. Aldose reductase is a key enzyme in regulating glucose level in non-insulin dependent diabetes mellitus, which increases substrate affinity toward glucose (Matsuda et al., 2017). The C2=C3 double bond in the flavone and flavonol subclasses might be an indicator of antiinflammatory effects due to its molecular planarity (Celik & Kosar, 2012;Wang et al., 2018).
Flavonoids have been known to have a significant potential to act as XO inhibitor. XO catalyzes the oxidation of hypoxanthine to xanthine. It also catalyzes the oxidation of xanthine to uric acid. This plays a very important role in gout. The accumulation of excess uric acid in serum leads to hyperuricemia. Hyperuricemia may lead to cardiovascular disease, gout, and other metabolic disorders (Hille, 1996;Borges et al., 2002). The possibility of exploiting the activity of natural dietary flavonoids as potential XO inhibitors in the treatment of such diseases has been emphasized in the literature (Panche, 2016). Licoisoflavone from Glycyrrhiza glabra roots, from which licorice is derived, was shown to have the strongest activity in XO inhibition (Anajjar et al., 2008). A Study performed by Lin et al., (2014) suggests that the flavone eupatilin, extracted from gnaphalium affine, showed stronger XO inhibitory effect, when compared to a synthetic XO inhibitor, allopurinol. Other flavones, such as apigenin and luteolin in gnaphalium affine extract, also acted as XO inhibitor. This study affirms the use of gnaphalium affine in treating gout. The XO inhibitory activity of the flavonoids might be related to aspects of their chemical structures that increase their binding affinity for XO. Lin et al. (2014) indicated that the planar structure and the C2=C3 double bonds of flavonoids such as flavones and flavonols promoted binding to XO and improved XO inhibition; on the other hand, hydroxylation on ring B was unfavorable for XO inhibition.
Flavonoids can be synthesized in plants following microbial infection. They are very effective substances in antimicrobial activities that act against an array of microorganisms. Flavonoids extracted from different plants show potent antimicrobial bioactivity against various microbes, including bacteria and viruses. Their ability to inactivate microbial adhesions, enzymes, and cell envelope transport proteins may determine the form of antimicrobial activities (Cowan, 1999;Panday et al., 2007;Mishra et al., 2013). It has been found that quercetin can fully stop the growth of Staphylococcus aureus. Staphylococcus aureus is a bacterium that may cause minor skin infections like acne or more serious diseases such as pneumonia (Havesteen, 1983) According to the study, flavonol compounds such as quercetin and kaempferol, which may be lipophilic, and polar flavonoids had the strongest antibacterial activity, and quercetin was a more effective inhibitor than luteolin. The two compounds show only one structural difference, which is that quercetin has a hydroxyl group at position 3 in the C ring but leteolin has none (Wu et al., 2013). This emphasizes the significance of the hydroxyl group at position 3 in the C ring on the antibacterial activity of flavonoids. This is conforming to the argument in previous studies that states the lack of a hydroxyl group at position 3 reduces the antimicrobial abilities of flavonoids (Sichel et al., 1991;Tripoli et al., 2007). Since the 1940s, many studies have also reported that naturally occurring flavonoids exhibit remarkable antiviral activity. Flavonoids play a part in inhibiting a variety of enzymes that are related to viruses’ life cycle. It has been noted that flavonoids’ ability to inhibit enzymes has a structural and functional relationship with flavonoids. Flavon-3-ol was proved to be a stronger inhibitor against two major types of human immunodeficiency virus (HIV), HIV-1 and HIV-2, than flavones and flavanones (Gerdin & Svensjo, 1983). Quercetin, morin, dihydroquercetin, and catechin was found to show antiviral effects as well. Their antiviral activity seems to have a relationship with the nonglycosidic compounds and hydroxylation at the 3 position. This may act as a prerequisite of the antiviral activity (Selway, 1986). According to a study by Thomas et al. (1988), flavonols with a hydroxyl group at the 3 position as well as nonglycosidic compounds were stronger than flavones against Herpes simplex virus (HSV) type I. Among the tested flavonols, galangin had the strongest antiviral effect, followed by kaempferol, and then quercetin. Additionally, a natural plant flavonoid polymer with a molecular weight of 2,100 Da was found to show antiviral activity against both HSV-1 and HSV-2, which produce viral infection in majority of humans (Loewenstein, 1979).
Cancer is a disease caused when cells grow uncontrollably. It is the third biggest killer in the world and its etiology is quite complex (Ahmadi & Shadboorestan, 2016). Cancer is a disease that goes through multistep processes and involves many factors, such as physical, environmental, metabolic, chemical and genetic elements. This complexity has a significant part in the initial stage of the disease. Additionally, oxidative stress can be crucial in the pathophysiology of different cancers (Ahmadi & Shadboorestan, 2016;Karak 2019). Changing one’s diet might be crucial in preventing many types of cancers. Flavonids representing phenolic compounds have been found to be major chemopreventive agents. They exhibit anticancer activity by modifying different signaling pathways. These pathways are involved in downregulating mutant p53 protein, cell cycle arrest, blocking cancer-causing enzymes, and expression of renin angiotensin system (RAS) proteins (Duthie et al., 2000;Mishra et al., 2013;Zhao et al., 2018). One of the most commonly found genetic abnormalities in human cancers are mutations in p53. Therefore, blocking the expression of p53 often results in the arrest of cancer cells. It has been believed that flavonoids are effective in downregulating mutant p53 protein expression, so that they are not detectable in human cancer cell lines. (Davis & Matthew, 2000). Tyrosine kinase is an enzyme found in the cell membrane and plays a role in the transduction of cell growth factor signals. Its expression may be involved in oncogenesis, as it may be capable of interfering with normal regulatory growth control. In conventional chemotherapy, tyrosine kinase activity is inhibited to prevent tumors. Based on the data of Boly et al. (2011), quercetin, a flavonol, was effective in modulating cells by acting through the protein kinase signaling pathway, even at low concentrations. Quercetin also works as an antineoplastic agent by initiating cell cycle arrest in proliferating lymphoid cells. Additionally, it showed positive results in inhibiting growth on several malignant tumor cell lines, such as leukemia cells, gastric cancer cells, colon cancer cells, human breast cancer cells, and ovarian cancer cells (Davis & Matthew, 2000). The C2=C3 double bond in quercetin is crucial for this strong tumor inhibition. However, there may be a greater effect when C2=C3 unsaturation and two ring B hydroxyl groups co-exist. Additionally, 3-hydroxylation of ring C may be highly important for improving these biological effects (Huang et al., 2010;Amrutha et al., 2014). The anticancer activity of flavonoids other than quercetin has been extensively reviewed by several studies. The anticancer effect of genisten, an isoflavone, in both in vivo and in vitro models has been reported by Barnes (1995). It was discovered that genistein suppresses the advancing of chemically induced mammary cancer without reproductive toxicity. Hesperidin, a flavanone glycoside, is also found to inhibit the development of azoymethane-induced colon and mammary cancer (Ren et al., 2003). In the earlier study, various flavonoid subgroups, including selected flavonols, flavones and flavanones along with isoflavone biochanin A, an O-methylated isoflavone, were known to show strong antimutagenic activity. Moreover, it was discovered that a carbonyl group at the C4 position of the flavone was necessary for this activity (Edenharder et al., 1993). It has been known for more than three decades that about a third of all human cancers, including pancreatic, lung, and colorectal cancers, are driven by mutations in RAS proteins. RAS proteins function as molecular signaling switches that are significant in normal cellular growth and differentiation, but a mutation in RAS proteins can be a fatal oncogenic factor due to the deregulation of normal cellular signaling pathways (Fernández-Medarde & Santos, 2011). Based on a study reported by Masoodi & Alhamdanz (2010), flavonoids such as naringenin, daidzein, and hesperetin showed the highest affinity to mutant RAS proteins without interacting with normal RAS proteins or producing cytotoxicity.
Summary
It is well known that phytochemicals like flavonoids can prevent and cure many chronic diseases of the human body. Flavonoids can be naturally sourced from fruits and vegetables. ROS free radicals are known to cause many chronic illnesses as mentioned earlier in this review. These exist in the human body as a result of various exogenous and endogenous causes. Considerable amount of research has shown the potential of plant-based flavonoids to support human health as a result of their ability to search for and neutralize ROS free radicals, which are responsible for the initial stage of cell injury. Flavonoids as phytochemicals constitute a major aspect of human health promotion and maintenance due to their functions as free radical scavengers, inhibitors of lipid peroxidation and xanthine oxidase, modulators of the inflammation response, and antimicrobial and anticancer agents. They are commonly called secondary metabolites in plants. They are often found among few taxonomic groups in plants (Croteau et al., 2000). Subdivisions of flavonoids are as follows: flavones, flavonols, flavanones, flavanols, isoflavones, and anthocyanins. They are molecules that have phenolic benzo-pyran structure. They are only found in plants and primarily exist as glycosides. A variety of flavonoids in nature possess unique chemical and physiological properties, and there is a strong correlation between their chemical structure and biological activities. Therefore, the biological activities of flavonoids are dependent upon their chemical structures, which are in turn dependent upon their structural class, level of hydroxylation, substitutions and conjugations, and level of polymerization. Generally, the position and number of hydroxyl groups in flavonoids correlate to their antioxidant activity. Hydroxyl groups in flavonoids function by donating hydrogens to ROS free radicals produced in biological systems, resulting in an antioxidant effect. Flavonoids also play a role as chelators of transition metals, which facilitate the formation of the hydroxyl radical, a powerful oxidant, in biological systems, resulting in the possible inhibition of lipid peroxidation. Trace metals are connected in certain positions on different rings of the flavonoid structure. The C2=C3 double bond present in flavones, flavonols, and isoflavones may confer antiinflammatory properties, which result in the potent inhibition of enzymes such as XO and COX-2, which are related to many chronic diseases. The hydroxyl group at position 3 of the C ring of flavonol has a strong correlation with the antimicrobial activities of flavonoids. Reports that the lack of the hydroxyl group at position 3 in flavonoids weakens their antimicrobial abilities strengthens this argument (Sichel et al., 1991;Tripoli et al., 2007). The existence of the C2=C3 double bond in flavonoids is essential for their anticancer activity, and when the double bond and two ring B hydroxyl groups exist together flavonoids inhibit cancer much more effectively. Finally, when the double bond is accompanied by 3-hydroxylation of ring C in flavonoids may be crucial for improving anticancer activity. Therefore, consumption of plant-based natural products such as flavonoids may be the best way to prevent and support the treatment of several chronic diseases. Although the sheer number and variability of plant-based flavonoids make the study of these metabolites difficult, their nutritional significance as well as their potential beneficial health effects warrant much more detailed studies. The ultimate goal of this research is to provide a basis for combining strategies to gain an understanding the function of dietary phytochemicals like flavonoids in preventing cancer, cardiovascular disease, and many other chronic illnesses caused by oxidative stress and the immune system. To fully grasp the importance of plant-based flavonoids, various factors must be taken into consideration. The bioavailability of flavonoids, their mechanisms and potential synergy effect with other elements should be investigated. Furthermore, further research is necessary in the content and composition of flavonoids in foods.