Introduction
Resveratrol (trans-3,5,4'-trihydroxystilbene) is a polyphenolic compound that is present in several plants belonging to the pineceae, mytaceae, fagaceae, moraceae, papilionaceae and vitaceae (Frémont, 2000). Resveratrol restricts platelet aggregation and coagulation, eicosanoid synthesis modification, and lipoprotein metabolism modulation. These unique properties have been shown to affect the treatment of heart disease as well as anti-cancer, anti-inflammatory, anti-oxidative, antibiotic and anti-aging activities (Frankel et al., 1993; Jang et al., 1997; Pinto et al., 1999). However, resveratrol is limited in applications that involve interaction with external environment such as air, light, and oxidative enzymes due to high hydrophobicity, poor stability and solubility. It exists in two isomeric forms cis and trans and have ability to convert into cis from trans isomer; unfortunately only trans-resveratrol has biologically activity. But trans-resveratrol has limited stability under the influence of light, certain pH levels and temperature, which can cause degradation or isomerization (Zupancic et al., 2015).
At present, one promising nanotechnology is the application of a delivery system containing nutraceutical ingredients with nanometer-sized emulsion form, which offers the potential to improve the solubility and bioavailability of many bioactive ingredients such as carotenoids, polyunsaturated fatty acids, phytosterols, antioxidants, vitamins, minerals and other natural compounds (Kim et al., 2009). Nanoemulsions can be prepared by self-assembly, which can be used to introduce guest molecules having hydrophilic, lipophilic, or amphiphilic characteristics. It is thermodynamically stable and isotropic in form, having a drop size less than 100 nm. It provides ultralow interfacial tensions and large oil-in-water (O/W) interfacial areas, so it has a higher solubilization capacity than simple micellar solutions, and their thermodynamic stability offers advantages over unstable dispersions, such as emulsions and suspensions (Lawrence & Ress, 2000). The stability of the nanoemulsion depends on the preparation method as well as the order in which its components were added in self-assembly emulsification (Wang et al., 2008). Self-assembly emulsification is accomplished using an isotropic mixture of oil, surfactant, and co-surfactant. Together, these form fine O/W emulsions upon introduction into aqueous media under agitation. The self-assembled structures that formed in the oil phase at a certain dilution composition can be hydrated with water to produce bicontinuous structures inverting into an O/ W structure (Garti et al., 2005).
Recently, food nanotechnology has focused on nanoencapsulation of bioactive ingredients such as capsaicin and resveratrol as well as the successful application of nanoemulsions (Cho et al., 2008; Kim et al., 2008). A bioactive ingredient can be encapsulated in nanometer-sized structures, which are transparent in form with sizes of 20-500 nm that provide solubility, stability, and bioavailability (Choi et al., 2008). Very few examples of nanoemulsion systems based on food-grade surfactants such as ethoxylated sorbitan esters (Tweens® ) and sucrose esters exist (Choi et al., 2009). Medium-chain triacylglycerols (MCTs) provide different chemical and physiological properties compared to unsaturated long-chain triacylglycerols (LCTs) such as soy, corn, cotton, and sunflower oil. Compared to LCTs, MCTs such as palm and coconut oil are fully saturated. The smaller size, lower melting points, and higher water solubility allows them to be stable in aqueous phase in response to oxidative rancidity at room temperature (Jeukendrup & Aldred, 2004). As such, MCTs are now utilized in many food applications, and they have been approved as GRAS (Generally Recognized as Safe) for oral or enteral use by the U.S. Food and Drug Administration (Traul et al., 2000). A simple solution or nanoemulsion may be unstable or subject to degradation when used as a food delivery system due to the inherent structure of the emulsion as well as environmental conditions such as temperature, light, and pH. It is possible to develop nanoparticles as novel delivery systems using food-grade biopolymers such as polysaccharides (alginate, chitosan, pectin, gum arabic) and proteins (casein, gelatin, whey) by promoting self-association or aggregation of single biopolymers or by inducing phase separation in mixed biopolymer systems (Kim & Karin, 2008). Therefore, single-layer nanoemulsions were named O/W nanoemulsions layered with aqueous phase based on water, oil phase, and surfactant. Double-layered nanoemulsions were named O/W nanoemulsions layered with alginate, chitosan and β-cyclodextrin in aqueous phase.
Currently, there are many different types of delivery systems for resveratrol. Such systems involve the incorporation of resveratrol into yeast cells, liposomes, calcium-pectinate or chitosan for controlled delivery as microspheres or nanospheres (Caddeo et al., 2008; Shi et al., 2008; Das & Ng, 2010; Peng et al., 2010). Although many researchers have different technologies about preparing resveratrol nanoemulsions, most of them used a high energy emulsification method such as high speed homogenization and ultra sound sonication. So we have proposed a low-energy emulsification method using ethanol in oil phase and a method of preparing nanoemulsions containing more than 100 μg of resveratrol with stable system. The objective of this study was to investigate the formulating conditions of single- and double-layers nanoemulsions containing resveratrol that are produced by self-assembly and complex coacervation using biopolymers such as chitosan, alginate and β-cyclodextrin. Furthermore, the characteristics and stabilities of the resveratrol nanoemulsions were evaluated to demonstrate the potential of resveratrol as a nutraceutical delivery system.
Materials and Methods
Chitosan was prepared by demineralization, deproteinization, and deacetylation from crab shell. Next, the chitosan (degree of deacetylation 93%, molecular weight 330,000) was dissolved in a mixed aqueous solution of 20% hydrochloride solution and distilled water, after which the mixture was adjusted to pH 5.6 and stirred for 3 h. The reaction mixture was then subjected to a continuous membrane filtration process, after which a permeate was freeze-dried (Seo & Toshio, 2002). Resveratrol, alginate sodium salt, β-cyclodextrin, and polyoxyethylene sorbitan monooleate (Tweens® 80) were purchased from Sigma Chemical Co. (St. Louis, MO, USA). MCTs (medium-chain triacylglycerols) were purchased from Wellga Co. (Seoul, Korea). Deionized distilled water was purchased from Waters (Milford, MA, USA). The R-(+)-limonene was purchased from Tokyo Kasei Kogyo Co. (Tokyo, Japan). Soybean oil, corn oil and canola oil were obtained from Baeksul Co. (Seoul, Korea). Olive oil was purchased from Sajoheapyo Co. (Seoul, Korea).
Chitin was prepared by treating Chitinonecetes opilio with 15% hydrochloride solution at 50°C for 24 h (demineralization) and, subsequently, with 5% sodium hydroxide at 90°C for 12 h (deproteinization). Then chitin was treated with 60% sodium hydroxide at 140°C for 24 h to remove the acetyl residues (deacetylation). Next, the chitosan (degree of deacetylation 93%, molecular weight 330,000) was dissolved in a mixed aqueous solution of 20% hydrochloride solution and distilled water, after which the mixture was adjusted to pH 5.6 and stirred for 3 h. The reaction mixture was then subjected to a continuous membrane filtration process, after which permeate was freeze-dried.
To measure resveratrol solubility, various oil phases were selected (MCTs, limonene, corn oil, soybean oil, olive oil, and canola oil). The selection of oil phase was evaluated with analysis of particle size, degree of turbidity, and separation of oil phase through visual observation on the preparation of the nanoemulsion. Therefore, the optimum oil phase was selected with MCTs that could manufacture particle sizes of less than 100 nm and transparent in form. After ternary mixtures consisting of various types and compositions of oil phases were pre-tested.
Resveratrol (10 mg) was added to 1 mL of ethanol, followed by the addition of the oil phases at a 1:1 ratio, respectively. The mixture was stirred for 5 min and then subjected to vacuum evaporation at 50°C to remove the ethanol. The oil phases and Tweens® 80 were then mixed together at a weight ratio of 1:3, followed by stirring for 5 min. To prepare the nanoemulsion, the mixture was accurately weighed (0.5 g) and diluted with deionized water (100 mL) as the aqueous phase, followed by magnetic stirring for 2 h and stabilization at 25°C for 24 h. Only with MCTs, measurement of the surfactant concentration was pre-tested using Tweens® 80 at different ratios (resveratrol +MCTs:Tweens® 80=1:1, 1:1.5, 1:2 and 1:3), based upon the results of our prior research involving the preparation of nanoemulsions using oleoresin capsicum (Choi et al., 2009). Tweens are widely used in food emulsion systems, primarily due to their relatively low toxicity, irritation potential and the ease with which they form micro- or nanoemulsions.
SLRNs consisted of oil droplets in an aqueous phase with the oil droplets being surrounded by a thin interfacial layer consisting of emulsifier molecules. SLRNs were prepared by self-assembly emulsification according to the following procedure. One of the six different oil phases used, MCTs produced highly dispersive emulsions consisting at all composition ratios of ethyl alcohol and resveratrol. Therefore, pre-mixture containing MCTs as the oil phase and Tweens® 80 as surfactant was selected. Resveratrol (10 mg) was added to 1 mL of ethanol, followed by the addition of MCTs at a 1:1 (v/ w) ratio. The mixture was stirred for 5 min and then subjected to vacuum evaporation at 50°C to remove the ethanol. The oil phase and Tweens® 80 were then mixed together at weight ratios of 1:1, 1:2, and 1:3 followed by stirring for 5 min (Table 1). To prepare the nanoemulsion, the mixtures were accurately weighed (0.5 g) and diluted with deionized water (100 mL). There is magnetically stirred for 2 h and stabilized at 25°C for 24 h. This primary emulsion was used for the preparation of SLRNs by dilution with deionized water.
Charged polyelectrolyte biopolymers are then added to the system of single layer nanoemulsions so that it absorbs to the droplet surface and produces DLRNs consisting of oil droplets coated by a biopolymer polyelectrolyte interface. DLRNs were prepared by complex coacervation with three different biopolymers: alginate (AN), chitosan (CN) and β-cyclodextrin (CYN). Alginate, chitosan and β-cyclodextrin solutions were prepared at concentrations of 0.05% in aqueous phase. Resveratrol (5 mg) was added to 1 mL of ethanol followed by the addition of MCTs at a 1:1 (v/w) ratio. The mixture was stirred for 5 min and then subjected to vacuum evaporation at 50°C to remove the ethanol. The oil phase and Tweens® 80 were then mixed together at a weight ratio of 1:2, followed by stirring for 5 min. This mixture was accurately weighed (0.5 g), diluted in each of the above biopolymer solutions instead of deionized water, and then stirred for 2 h at 25°C. The nanoemulsions were then filtered with a 0.45 μm PVDF membrane filter (Whatman, Maidstone, Kent, UK) and stabilized at 25°C for 24 h before analysis.
The particle size distributions of the nanoemulsions were determined by photon correlation spectroscopy which analyzes the fluctuations in light scattering due to the Brownian motion of the particle using a Nanotrac NPA 250 (Microtrac Inc., Montgomeryville, PA, USA). Light scattering was monitored at 25°C at a 90° angle. The zeta-potentials of the nanoemulsions were measured by a ZetaPlus (Brookhaven Instruments Corp., Holtsville, NY, USA), which measures the distribution of the electrophoretric mobility of the particles using laser light scattering. 1.6 mL of each sample was poured into the electrophoretic cell. The particle size distribution and zetapotential value was analyzed at the optimum concentration range of Nanotrac and ZetaPlus through diluting between 10 and 100 times with deionized water. Each measurement was repeated 5 times on three different samples of the same composition.
The storage stability of SLRNs and DLRNs were evaluated by measuring the particle size and zeta-potential value during storage at 25°C for 4 weeks. The determination methods of particle size and zeta-potential value were same as above described method.
The stability of resveratrol from the SLRNs and DLRNs was determined by measuring the amount of resveratrol using liquid-liquid extraction. In this study, only CYN was used in the release test of resveratrol from DLRNs. The initial resveratrol content of the nanoemulsions was designated as 100%, whereas the decreasing resveratrol content was calculated via HLPC for 7 storage days at 25°C. The liquidliquid extraction of resveratrol was performed as follows. Nanoemulsions (1 mL) were mixed with 100% MeOH (2.5 mL) for 1 min, followed by the addition of hexane (10 mL) with stirring for 3 min. This mixture was maintained for 10 min at room temperature prior to the extraction of resveratrol. The methanol layer was recovered using a micro-syringe and then filtered with a 0.45 μm syringe filter. The extracted resveratrol was then analyzed by HPLC using a gradient controller unit 601-602 (FLOM, Tokyo, Japan) with an injection volume of 20 μL. Separation was performed on a C18 column (Zorbax Eclipse XDB-C18, 4.6×250 mm, 5 μm; Agilent Technologies, Santa Clara, CA, USA). The mobile phase used was 100% acetonitrile, and the flow rate was 0.6 mL/min for 10 min. The UV/VIS detector S-3740 (Soma, Tokyo, Japan) was set at 308 nm. Standard curves were made using resveratrol dissolved in methanol (10, 30, 50 and 100 ppm).
All results are expressed as the mean±standard deviation. Statistical analyses of the data were carried out using SAS software (SAS Institute, Cary, NC, USA). Significant differences among groups of nanoemulsions were determined by Duncan’s multiple range test with p<0.05, p<0.01 and p<0.001, were considered statistically significant.
Results and Discussion
Resveratrol at a concentration of 5 mg/mL was solubilized in ethanol and then mixed with MCTs, limonene, corn oil, soybean oil, olive oil and canola oil at ratios of 1:1. These resulting mixtures were then added to Tweens® 80 at ratios of 1:3 (w/w). The emulsion was prepared by dissolving the mixtures with water at concentrations of 0.5%. When the ethanol-resveratrol solution was mixed with corn oil, soybean oil, olive oil, and canola oil, it became cloudy and separated from the oil phase. Additionally, the particle size was found to be within 191-936 nm (Table 2). In contrast, MCTs and limonene showed particle sizes of about 16.15 and 12.11 nm, respec- tively, which implies they can serve as the oil phase for the production of resveratrol-containing nanoemulsions. Resveratrol does not completely dissolve in buffering solutions with pHs of 4-5, but it does show relatively high solubility in coconut oil as well as glycerol (Hung et al., 2006). Additionally, the use of limonene is limited due to its characteristic flavor. Therefore, MCTs are considered to be the appropriate oil phase for the production of nanoemulsions containing resveratrol. In addition, due to its high solubility in ethanol, resveratrol could be used for the manufacture of nanoemulsions containing high concentrations of resveratrol.
Oil phase | Particle size(nm) |
---|---|
MCTs1) | 16.15±0.222)e4) |
Limonen | 12.11±0.77e |
Corn oil | 190.67±6.03d |
Soybean oil | 634.67±23.59c |
Olive oil | 764.67±12.58b |
Canola oil | 935.67±31.94a |
F-value | 1,651.02***3) |
Table 1 displays the particle sizes and resveratrol contents of nanoemulsions based on various ratios of resveratrol and MCTs with Tweens® 80. The highest resveratrol content (250 μg/mL) was produced when the resveratrol and MCTs mixture was mixed with Tweens® 80 at a 1:3 ratio (Resveratrol+MCTs : Tweens® 80 : water composition ratio of 5 : 15 : 80, w/w) (Table 1). In addition, when resveratrol and MCTs were mixed with Tweens® 80 at a 1:2 ratio, the particle size was 30 nm and the resveratrol content was 83.3 μg/mL (Resveratrol+MCTs : Tweens® 80 : water composition ratio of 1.67 : 3.33 : 95, w/w). The particle sizes of the nanoemulsions after mixing of resveratrol and MCTs with Tweens® 80 at ratios of 1:1, 1:1.5, 1:2 and 1:3 are presented in Table 3. When the mixing ratio was 1:2 or 1:3, the particle sizes of the nanoemulsions were 16.3 and 16.2 nm, respectively. However, mixing ratios of 1:1 and 1:1.5 resulted in sizes of 231 and 554 nm, respectively, which indicates that the emulsions must be unstable if subjected to storage. Based on the results, the appropriate mixing ratio of Tweens® 80 for the production of resveratrolcontaining nanoemulsions is considered to be two times that of the weight of the resveratrol and MCTs mixture. A phase diagram of the three-component resveratrol+MCTs/Tweens® 80/water system at ratios of 1:1, 1:1.5, 1:2 and 1:3 is presented in Fig. 1. This composition ratio of Tweens® 80 could be used as a quality indicator related to the allowance limit of additives in the food industry. The phase behavior of such samples was determined only after sharp interfaces become visible. When the mixing ratio of the resveratrol and MCTs mixture with Tweens® 80 was 1:2 or 1:3 in 60% water, nanoemulsions formed with particle sizes of less than 100 nm, which is indicated as the white region in the diagram. There were exhibited white areas as one-phase nanoemulsions region (below 100 nm) and gray areas as multiple-phase nanoemulsions (between 100 and 700 nm), respectively. Because nano-sized emulsions below 100 nm absorb light, these samples will have a transparent appearance. This differs from the micro-sized emulsions larger than 300 nm which will scatter light sufficiently to allow them to be seen by naked eye and have a cloudy white appearance.
Mixture ratio Res+MCTs:T1) | Particle size(nm) |
---|---|
01:01.0 | 554.00±112.942)a4) |
01:01.5 | 230.87±23.53b |
01:02.0 | 16.29±0.47c |
01:03.0 | 16.15±0.22c |
F-value | 58.23***3) |
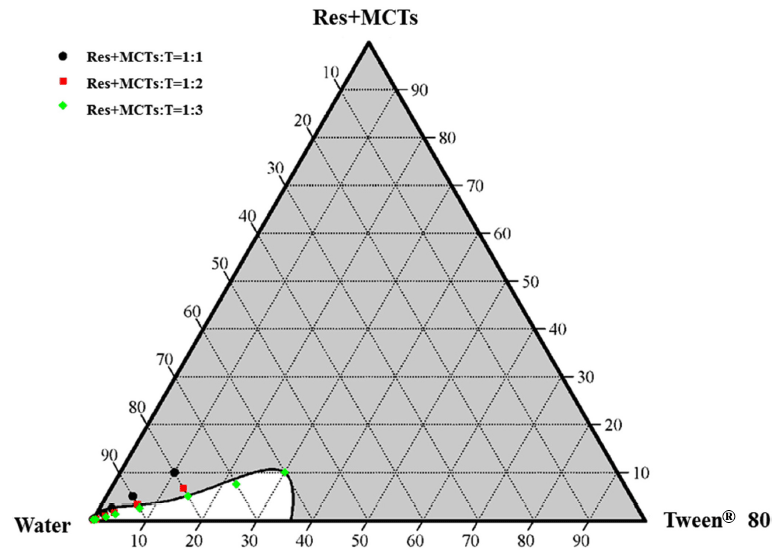
To confirm the stability of SLRNs, the mixing ratios of the resveratrol and MCTs mixture with Tweens® 80 were varied as 1:1, 1:2 and 1:3, and the particle sizes of the resveratrol nanoemulsions during 4 weeks of storage at 25°C were measured (Table 4). When the mixing ratio was 1:1, the particle sizes of emulsions were 136-176 nm at mixture concentrations of 0.5, 1 and 3% (w/w); whereas, the particle sizes of 251-655 nm were observed at concentrations of 5, 10, and 20% (w/w) at 0 day. When the mixing ratio was 1:2, particle sizes of 27, 25, 24, and 30 nm were observed to be concentrations of 0.5, 1, 3, and 5%, respectively, showing that particle size was reduced by increasing the amount of Tweens® 80 and maintaining a stable state during storage. A mixing ratio of 1:3 resulted in particle size of less than 15 nm when the Tweens® 80 was added at concentrations of 0.5, 1, 3, 5, and 10% (w/w); these nanoemulsions were stable throughout storage. For the production of nanoemulsions, the amount of Tweens® 80 added has large effects on particle size reduction and stability of the nanoemulsion (Choi et al., 2009). The storage stabilities of the resveratrol nanoemulsions prepared by mixing resveratrol and MCTs with Tweens® 80 with a ratio of 1:2 are presented in Table 5. The DLRNs prepared with alginate and chitosan were unstable and showed slight increases in particle size during the 4 week storage period. However, the DLRNs prepared with β-cyclodextrin were more stable with particle sizes of less than 40 nm during storage. For the SLRNs, they were relatively stable during storage, but their stability was slightly reduced after 2 weeks of storage. These differences in stability between the SLRNs and DLRNs may be due to their initial particle sizes governed by their composition that included surfactants, bio-polymers (e.g. alginate and chitosan), and lipids.
1) Res+MCTs:T=1:1 : resveratrol+medium-chain riaylglycerols:Tween®80=1:1, 2) Mixture:Water : Resveratrol+MCTs+Tween®80:Water
1) SLRN: single-layer resveratrol nanoemulsion based on water, CN: double-layers resveratrol nanoemulsion prepared by complex coaervation with chitosan, AN: double-layers resveratrol nanoemulsion prepared by complex coaervation with alginate, CYN: double-layers resveratrol nanoemulsion prepared by complex coaervation with β-cyclodextrin
The droplets in most nanoemulsions have an appreciable electrical charge; therefore, electrostatic interactions may play an important role in determining their overall stability and physicochemical properties. The zeta potential, which represents the electric charge between the shear plane of the final external layer and the bulk solution, has been used to estimate the property of dispersions. The zeta potentials of the resveratrol nanoemulsions prepared by mixing resveratrol and MCTs with Tweens® 80 at a ratio of 1:2 are indicated in Table 6. The zeta potential of the DLRN prepared with chitosan was 0-37.7 mV during storage due to the positive charge of the chitosan molecules coated on the surface of nanoemulsions. In contrast, the zeta potential of the DLRN prepared with alginate was -14.4 - -24.8 mV due to the negative charge of the alginate molecules. Meanwhile, the zeta potential of the DLRN prepared with β-cyclodextrin resulted in a stable negative value ranging from -5.6 - -14.6 mV. The droplets of most nanoemulsions have an appreciable electrical charge, suggesting electrostatic interactions may play an important role in determining overall stability and physicochemical properties (Nielloud & Marti-Mestres, 2000). The zeta potential represented by the electric charge between the shear plane of the final external layer and the bulk solution, is used to estimate the extent of dispersion. Furthermore, this factor is influenced by the electrical characteristics between nanoemulsion particles.
1) SLRN: single-layer resveratrol nanoemulsion based on water, CN: double-layers resveratrol nanoemulsion prepared by complex coaervation with chitosan, AN: double-layers resveratrol nanoemulsion prepared by complex coaervation with alginate, CYN: double-layers resveratrol nanoemulsion prepared by complex coaervation with β-cyclodextrin
The stability of resveratrol from the DLRN prepared with β- cyclodextrin is shown in Table 7. The resveratrol content of the DLRN prepared with β-cyclodextrin was much higher than the SLRN, which it was reduced to 94.6% after 7 days. However, for the SLRN, the content of resveratrol was reduced to about 88.5% in the same storage period, demonstrating lower stability compared to the DLRN. This result indicates that delayed resveratrol release when resveratrol was present in an emulsion or aqueous micelle system. Moreover, the stability of resveratrol is related with its reduction in the order of aqueous micelle system > tocol emulsion > lipid emulsion (Hung et al., 2007). Therefore, the DLRNs prepared with β- cyclodextrin have potential as a nutraceutical delivery system for the purpose of increasing the stability and utility of physiological active compositions obtained from functional foods within the body. Controlled release of resveratrol is dependent on pH with slower release kinetics at higher pH values due to the protonated amino groups of chitosan resulting in a soluble, positively-charged polysaccharide that causes fast swelling in acidic medium (Peng et al., 2010).
Sample | Storage (day) | |||||
---|---|---|---|---|---|---|
0 | 5 | 7 | F-value | |||
SLRN1) | 100a | 92.09±3.412)b4) | 88.48±4.10Mb | 10.99**3) | ||
CYN | 100a | 98.82±0.27b | 94.58±0.56c | 187.48*** |
The biopolymers such as chitosan and alginate were increased the stability of resveratrol via complexation with DLRNs. However, the DLRNs prepared with β-cyclodextrin resulted in the highest stability. Therefore, β-cyclodextrin was the best in maintaining the stability of the resveratrol nanoemulsions. In addition, β-cyclodextrin is available in solid and powder form, making it ideal in a nutraceutical delivery system.
Summary
Resveratrol nanoemulsions prepared with chitosan, alginate, and β-cyclodextrin were obtained with particle sizes of 10-800 nm, depending on the emulsification parameters such as the ratio of aqueous phase and surfactant. Resveratrol nanoemulsions maintained their particle size and zeta-potential value during storage for 28 days at 25°C. The double-layers resveratrol nanoemulsions prepared with alginate or chitosan showed slight increase in particle size from 45 to 166 nm during storage period. The double-layers resveratrol nanoemulsions prepared with β-cyclodextrin relatively stable with a particle size of less than 40 nm. Stability of resveratrol in the double-layers nanoemulsion prepared by complex coaervation with β-cyclodextrin was higher than single-layer nanoemulsion, maintained approximately 95% of resveratrol content until 6 days of storage. Consequentely, the double-layers of resveratrol nanoemulsion prepared with β-cyclodextrin showed the best stability of the resveratrol having particle sizes below 100 nm. Further, β-cyclodextrin is available in solid and powder form, which makes its use ideal in a nutraceutical delivery system for increasing solubility, stability, and bioavailability of functional components.