Introduction
Anthocyanin is a class of natural pigment molecules typically found in flowers, leaves, and stems of trees as well as in fruits. Anthocyanins produce red, purple and blue colors depending upon the molecular structure and pH (He & Giusti, 2010).
Chemically, anthocyanins are sugar-modified (glycosylated) version of anthocyanidins. The sugar-free anthocyanidins are main structure responsible for the color formation of anthocyanins (Castañeda-Ovando et al., 2009).
According to the recent trend of avoiding artificial compounds in food coloring, anthocyanins are gaining increasing attention as the natural source of food coloring (Francis, 1989). In addition, the potent anti-oxidant activity of anthocyanin molecules are investigated as therapeutics as well as food additives with potential benefits against a variety of diseases including cancer (Sehitoglu et al., 2014) and inflammatory bowel diseases (Sodagari et al., 2015).
In this regard, maintaining the stability of anthocyanin structures during food production and processing is becoming an important subject. Different anthocyanidins and their sugarmodified anthocyanins have been tested for their stability upon heat and pH (Castañeda-Ovando et al., 2009). Several studies have shown that the functional groups attached to the core anthocyanidin structure affects stability of the molecule and its color.
The sugar modification has been considered as a critical determinant of anthocyanin stability. Previous studies have shown that sugar-modification of anthocyanidin confers stability to the core molecule (Osami et al., 2009; Zhao et al., 2014). However, a recent study reported that sugar-modification might have an adverse effect of the core anthocyanidin stability (Rakić et al., 2014). Therefore, further studies are warranted to clarify the role of sugar modification on the structural stability of anthocyanidin core molecule.
Anthocyanins are the main color-producing components in extracts of red cabbage. A previous study reported the composition of anthocyanins in red cabbage using liquid chromatography mass-spectrometry. The predominant anthocyanins were cyanidin-3-diglucoside-5-glucosides with diverse acylation patterns (McDougall et al., 2007).
In this study, we tested the thermal stability of the color of naturally extracted cyanidin derivatives from red cabbage and compared it with those of unmodified cyanidin and cyanidin- 3,5-diglucoside structures. Our observation supports the idea that sugar modification generally confers stability to the core anthocyanidin molecular structure.
Materials and Methods
Anthocyanin mixtures from red cabbage (Cat # L301, MSC Corp., Busan, Korea) and purple sweet potato (Cat # L509, MSC Corp., Busan, Korea) are purchased and prepared as a stock at 50.0 mg/L solution (pH 5). Baking powder (Arm & Hammer, Princeton, NI, USA) is used to make basic solution (pH 9). As a controls, Cyanine Chloride (Cas # 528-58-5 ChromaDex, Irvine, CA, USA) and Cyanine 3,5-diglucoside (Cas #2611-67-8, ChromaDex, Irvine, CA, USA) were purchased and prepared as a stock at 50.0 mg/L solution (pH 5).
To test the heat stability, the lowest color changing temperatures were visually observed using anthocyanin mixtures from red cabbage and purple sweet potato and 2 control anthocyanins (Cyanine Chloride and Cyanine 3,5- diglucoside). Using heat block, 1/100 diluted anthocyanins from the stock solution (50.0 mg/L in stock and 0.5 mg/L in working) were incubated for 10 min at each temperature. Temperature range for incubation is used from 25℃ to 65℃. To make sure the anthocyanins heat-treated fully, 10 micro liter of anthocyanin solutions in 0.7 mL tube are used.
To quantitate the color change, UV absorbance is measured for anthocyanin mixtures after heat treated at each different temperature. Spectrometer (Ultra Spec 7000, GE Healthcare, Pittsburgh, PA, USA) is used for scanning the wavelength from 400 nm to 700 nm of solution and analyzing the data after incubation.
Result and Discussion
A common structure of red cabbage anthocyanins are shown in Fig. 1A. A previous study demonstrated that the major anthocyanin components from red cabbage are cyanidin-3- diglucoside-5-glucosides (Fig. 1B). To compare the thermal stability and the role of sugar modification on thermal stability of cyanidin, we studied two additional molecules with core cyanidin structures, such as unmodified cyanidin (Fig. 1C) and cyanidin-3,5-diglucoside (Fig. 1D). In addition to the red cabbage, the major component of anthocyanins in purple sweet potato is glycosylated cyanidins (Montilla et al., 2011) Therefore, we included anthocyanins from purple sweet potato as another glycosylated form of cyanidin for stability experiments.
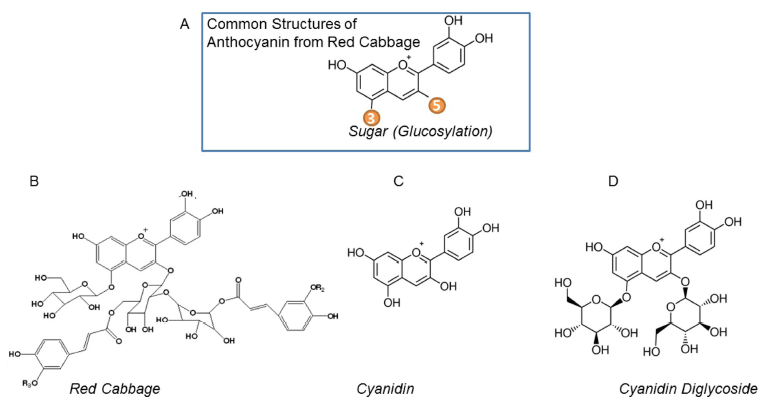
Thermostability of various red cabbage anthocyanin structures were investigated in acidic condition (pH 5) by incubating in different temperatures and visually observing the color disapprearance and also by analyzing the absorption spectra. As expected, the anthocyanins from red cabbage (Fig. 1B) did not show remarkable color change in any temperature tested based upon the UV absorbance spectra (Fig. 2A). Visual inspection also confirmed UV spectra observation (Fig. 2B).
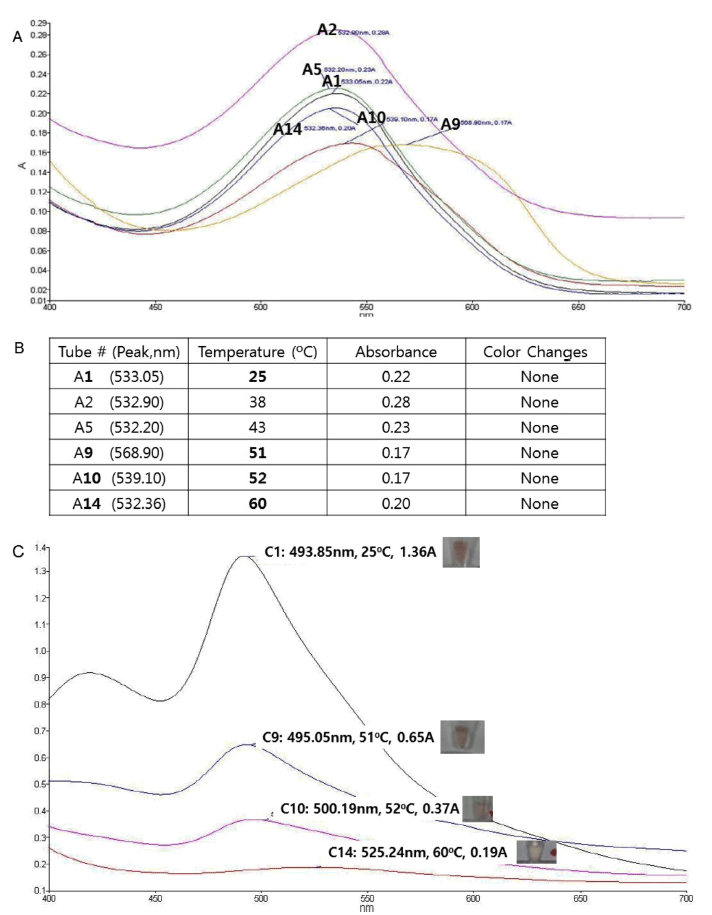
In contrast, unmodified cyanidin (Fig. 1C) showed color change at 58 to 60 ℃ in acidic condition as observed by both UV spectra measurement and direct visual inspection (Fig. 2C), showing that the core cyanidin structure is thermosensitive, and the modification of cyanidin confers thermostability of red cabbage anthocyanins.
To test whether the sugar modification confers thermostability to the core cyanidin structure, we tested the color change of cyanidin-3,5-diglucoside (Fig. 1D) upon temperature change in acidic condition. As shown in Table 1, cyanidin-3,5- diglucoside did not change its color upon heating, demonstrating that the glucoside modification on both 3 and 5 position of cyanidin can confer the thermostability.
As summarized in Table 1, this trend was also observed in basic condition (pH 9). In basic condition, unmodified cyanidin and cyanidin-3,5-diglucoside were somewhat less stable than in acidic condition, as the color-changing temperatures were decreased compared with acidic condition.
Nonetheless, the order of stability (cyanidin from red cabbage > cyanidin-3,5-diglucoside > cyanidin) remained same as in acidic condition.
Rakić et al. (2014) showed that cyanidin-3-glucoside reduces thermal stability of core anthocyanidin molecule. In our study, however, we observed that cyanidin-3,5-diglucoside confers thermostability compared with unmodified cyanidin. The main difference is the sugar modification at 5-position of core cyanidin molecule. Further studies are warranted whether the position 5 modification plays a key role in thermostability of anthocyanins.
In summary, our study confirms and further supports the idea that sugar modification stabilizes core anthocyanidin structure against thermal exposure. We believe that this finding will help manufacturers develop stable, natural pigments which can be utilized for safe food coloring and other medical application of anthocyanins.
Conclusion
We compared the thermal stability of color from unmodified cyanidin and cyanidin-3,5-diglucoside with the color of cyanidin derivatives from red cabbage. While it has been generally believed that glucoside modification increases anthocyanin thermal stability, a previous study reported that cyanidin-3-glucoside reduces thermal stability of core cyanidin molecule. In contrast to their study, however, our study shows that glucoside modification of cyanidin (cyanidin-3,5-diglucoside) confers thermostability compared with the unmodified cyanidin. Therefore, our results clearly demonstrate that the glucoside modification increases the thermal stability of cyanidin core molecule.