Introduction
In recent years, various studies have been conducted to distinguish α-glucosidase inhibitors from natural resources. Inhibition of the α-glucosidase, involved in the digestion of carbohydrates, can delay the release of α-glucose from disaccharides and oligosaccharides (Hong, 2011). A considerable number of synthetic substances that can potentially alleviate the condition of diabetic patients have been reported, although they result in several gastrointestinal complications. For example, acarbose, which reduces postprandial blood sugar levels and insulin sensitivity, results in side effects such as diarrhea and bloating (Liu et al., 2019). As an alternative to such synthetic products, inhibitors from natural product, and many plant materials have been developed as secondary metabolites including flavonoids, alkaloids, and terpenoids (Assefa et al., 2020).
In the last few decades, the soybean components that have received significant attention are isoflavones, which are polyphenols that contain approximately 0.2-0.4% of isoflavone derivatives such as isoflavone glucosides (malonyldaidzin, malonylgenistin, daidzin, glycitin and genistin) and isoflavone aglycones (daidzein, glycitein and genistein) (Setchell at al., 1998;Rizzo et al., 2018). Isoflavones in soybean primarily exist as glycosides wherein a peripheral glycosidic linkage bind them to a sugar moiety (Murphy et al., 2002;Nakajima et al., 2005). However, during food fermentation, due to various processes or gut digestion, this glycoside linkage is broken via the action of β-glucosidase enzymes resulting in the formation of isoflavone aglycones (Murphy et al., 1999;Otieno et al., 2007;Yamabe et al., 2007). The malonylglucoside form of isoflavone is predominant among soybean, however, a minor fraction of isoflavone occurs in the acetylglucosides or the aglycone form of isoflavone after thermal treatment (Chiarello et al., 2006;Mathias et al., 2006). Izumi et al. (2000) reported that isoflavone aglycones (daidzein and genistein) play a significant role in maintaining the higher concentration of isoflavones in plasma, as compared isoflavone glycosides (daidzin and genistin).
Previously, Shukla et al. (2015) reported that the α- glucosidase inhibitory activities of water-soluble extracts of fermented soybean paste samples at 5-50 mg/mL increased in a dose-dependent manner. These results suggest that higher α- glucosidase inhibitory activities could be due to an increase in the overall content of phenolic compound, which is one of the various bioactive phytochemicals in soybean. Tadera et al. (2006) reported that the α-glucosidase of yeast was potently inhibited by standard isoflavone aglycones, such as daidzein and genistein, with IC50 values of less than 15 μM. Similarly, Ha et al. (2019) reported that the chemical form of isoflavone aglycone (daidzein and genistein) inhibited α-glucosidase with an IC50 lower than 15 μM, whereas the chemical forms of isoflavone glycoside and isoflavone malonylglucosdie inhibited α-glucosidase with an IC50 higher than 100 μM.
The profile and content of isoflavone in soybean can be altered by factors such as heat, energy, and moisture content (Huang et al., 2014). In this respect, blanching, which involves thermally treating raw food materials with hot water at 82- 93°C within 3 min, and cooking, which take place at 95°C for 30-60 min (Toda et al., 2000;Noh et al., 2006), are both wellestablished food processing methods. In this study, either blanching or cooking was chosen for the thermal treatment of soybean, followed by the preparation of soybean extract from the respective blanched or cooked soybean. Finally, the isoflavone profiles and α-glucosidase inhibitory activities for these treated soybean extracts were examined to determine the effect of thermal treatment on soybean.
Materials and Methods
Commercially available soybeans were obtained from a domestic soybean-manufacturing agricultural facility located in Miryang. Acetonitrile, acetic acid and methanol (HPLCgrade) were purchased from Daejeong Chemical (Siheung, Korea). Standard isoflavones with 99% purity (daidzin, glycitin, genistin, daidzein, glycitein, genistein) and rat intestinal acetone powder (RAP) were purchased from Sigma-Aldrich (Woburn, MA, USA). 6"-Malonyl-glucoside was purchased from Nagara Science (Nagoya, Japan). p-Nitrophenyl-α-Dglucopyranose (pNPG) was purchased from Merck Millipore (Darmstadt, Germany).
Soybean extracts were prepared under different thermal treatment condition (Toda et al., 2000;Noh et al., 2006). Raw soybean extracts (RSe) were prepared by extracting raw, ground soybean using 80% ethanol. Blanched soybean extracts (BSe) and cooked soybean extracts (CSe) were obtained by 80% ethanol extraction of ground soybean pre-treated with hot water at 85°C for 3 min and 95°C for 30 min, respectively. A flow chart describing the preparation of soybean extract samples is shown in Fig. 1.
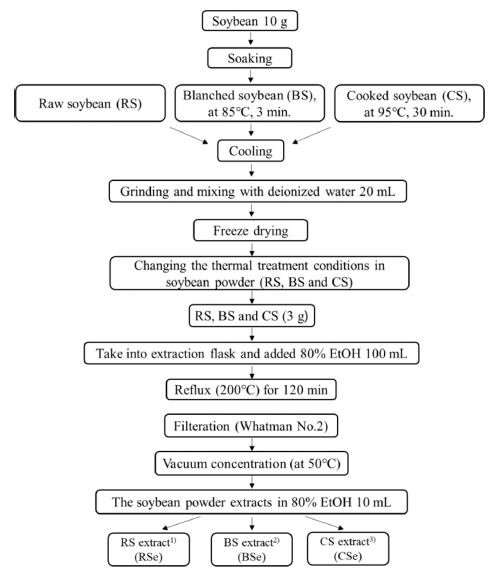
High performance liquid chromatography analysis of isoflavones followed the procedure described by Silva LH et al. (2011) and is summarized in Table 1. The analysis was carried out using an ACME 9000 HPLC system (Youngincm Co., Ltd., Anyang, Korea) comprising an Autochro-3000 data system, ACME 9000 pump, ACME 9000 column oven, and a UV detector. A Shim-pack GIS C18 column (250 mm × 4.65 μm) (Shimadzu Co., Kyoto, Japan) was used at an oven temperature of 30°C. Chromatograms for quantitative analysis were extracted at 254 nm. The samples were eluted at 0.5 mL/ min with a linear gradient of 5.0% (v/v) acetic acid in water (A) and methanol (B). The content of isoflavone glucoside was converted into the content of isoflavone aglycone by multiplying with the conversion coefficient (1/1.6) offered in the health food code (MFDS, 2018).
The α-glucosidase inhibitory activities of the isoflavones and soybean extracts were determined according to a method described by Lee et al. (2014). In a typical example, RAP (30 mg/mL) was initially homogenized in ice-cold 50 mM potassium phosphate buffer (4 mL). The clear supernatant obtained was used as the enzyme solution after centrifugation at 4000 rpm for 10 min. Soybean extract (100 μL) was added to the wells of a 96-well plate, followed by a suspension of RAP (50 μL), 3 mM pNPG (100 μL) as a substrate, and distilled water (50 μL). The mixture was then incubated at 37°C for 60 min to produce 4-nitrophenol. The reaction was terminated by adding Na2CO3 (750 μL, 0.1 M). The formation of 4-nitrophenol in each well was measured based on the absorbance at 405 nm using a microplate reader (BKMPR- 1101, Konvision Inc., Gwacheon, Korea). The control and the blank groups excluded the sample and pNPG, respectively. Acarbose inhibited rat small intestinal α-glucosidase with an IC50 of 613 μM, which was similar to that (613-620 μM) reported by Ha et al. (2019).
Data are presented as the mean standard deviation of triplicate values. Statistical significance was calculated using the Student’s t-test and one-way analysis of variance (ANOVA) in SPSS software (Statistical Package for the Social Sciences, Version 21.0, SPSS Inc., Chicago, IL, USA). Statistical significance was set at p<0.05.
Results and Discussion
The isoflavone profiles of the thermally treated soybean extracts were analyzed (Fig. 2) and their isoflavone compositions were quantified (Table 2). Approximately 88.4% of isoflavones in RSe, prepared without thermal pretreatment of soybeans, were found in the form of isoflavone malonylglucosides and isoflavone glucosides. Isoflavone aglycones accounted for 11.6% of RSe. Although this result was somewhat different from a previous report claiming that 97.3% of isoflavones in unprocessed soybean extract are in the glucoside form (Silva et al., 2011), the isoflavone composition may vary according to the variety of soybean. In addition, the difference in the amount of isoflavone glucosides between RSe and unprocessed soybean extracts could be attributed to their distinct preparation conditions.
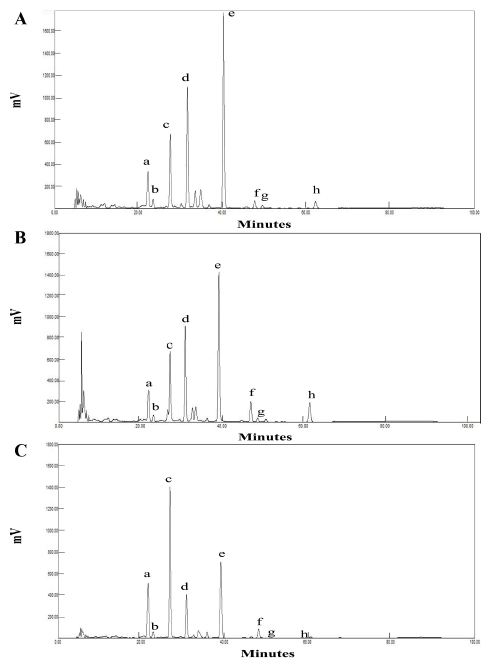
Malonylglucosides such as genistein 7-O-(6"-O-malonyl) glucoside and daidzein 7-O-(6"-O-malonyl)glucoside, occur at a relatively higher concentration in RSe. For example, the concentration of isoflavone malonylglucosides in RSe was 190.7±0.2 μg/g as compared to a lower concentration of 157.6±0.6 μg/g in BSe and a substantially lower concentration of 77.3±0.8 μg/g in CSe (Table 2). It was also noted that on changing the parameters for the thermal treatment of soybeans, such as from no thermal treatment to blanching (85°C, 3 min) and cooking (95°C, 30 min), there was a noticeable decrease in the concentration of isoflavone malonylglucosides in BSe and CSe, as compared to RSe. The decrease in the isoflavone malonylglucosides in BSe and CSe resulted in a corresponding increase in the concentration of isoflavone glucosides or aglycones. Meanwhile, the content of isoflavone glucosides in RSe was 67.8±0.1 μg/g as compared to that of 78.0±0.8 μg/ g in BSe and 123.1±1.0 μg/g in CSe. Unlike the isoflavone malonylglucosides, the isoflavone glucosides gradually increased in concentration in both BSe and CSe. The higher amount of isoflavone glucosides could be attributed to the thermal transformation of isoflavone malonylglucosides. Nevertheless, there was not much difference in the total content of converted isoflavones between RSe and BSe, but there was a significant difference between RSe and CSe. Based on these observations, it can be concluded that the cooking treatment in this study had a considerable impact on isoflavone malonylglucosides. Eventually, one quarter of isoflavones in the RSe was depleted during the thermal treatment transformation to CSe.
When it comes to the content of isoflavone aglycones (21.3±0.9 μg/g) in RSe, it increased considerably to 31.9±0.9 μg/g in BSe but decreased to 15.3±0.8 μg/g in CSe. This observation implied that a portion of isoflavone glucosides in both BSe and CSe was converted to isoflavone aglycones during the thermal treatment of soybeans prior to their extraction. Lee et al. (2014) have similarly reported that the isoflavone glucosides of soybean were converted, to a certain degree, into their corresponding isoflavone aglycone counterparts by thermal treatment and the conversion rate seemed to be affected by thermal treatment conditions, such as temperature and time. Kim et al. (2021) also reported that the glycoside isoflavones were efficiently converted into aglycones and confirmed an increasing aglycone content with increasing treatment time during steaming of soybean.
The α-glucosidase inhibitory activity of standard isoflavones is shown in Fig. 3. Authentic isoflavone aglycones of daidzein (54.4%), glycitein (66.9%) and genistein (77.1%) exhibited relatively significant inhibiting effects on the α-glucosidase, compared to the corresponding isoflavone glucosides of daidzin (35.6%), glycitin (48.7%) and genistin (39.8%). In addition, the inhibitory effects (47.1% and 50.7%, respectively) of malonyldaidzin and malonylgenistin were not comparable to those of the corresponding aglycones, daidzein (54.4%) and genistein (77.1%).
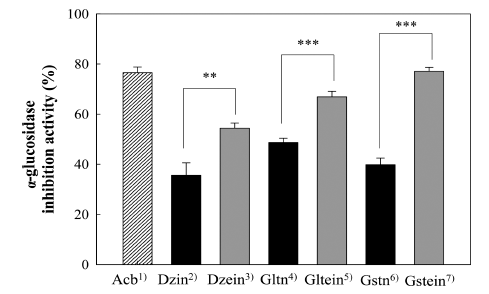
In this study, isoflavone aglycones were found to be more effective in the inhibition of α-glucosidase as compared to the corresponding isoflavone glucosides. These results complement the fact that isoflavone aglycones showed lower IC50 values for the inhibition of α-glucosidase than isoflavone glucosides (Ha et al., 2019). In a related study by Murota et al. (2002), a more than 10-fold higher transport of isoflavone aglycones (daidzein and genistein) during the intestinal absorption of purified isoflavone by Caco-2 cell monolayers was observed in comparison to corresponding isoflavone glucosides (daidzin and genistin). The inhibitory potential of the other flavonoids against α-glucosidase was also investigated (Fig. 4). For example, the inhibitory effect (54.0%) of quercetin on α- glucosidase was found to be much higher than that of rutin (quercetin-3-rutinoside) (31.6%), which implied that the aglycone of ordinary flavonol would similarly have a greater effect on α-glucosidase than the glucoside of the flavonol.
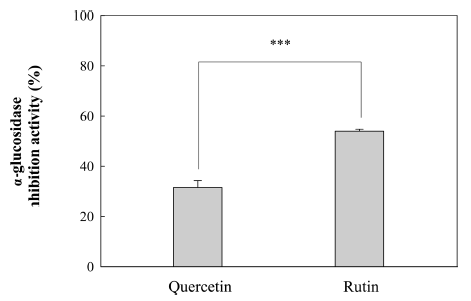
The α-glucosidase inhibitory activities of soybean extracts were as follows: BSe (70.0%), CSe (53.3%) and RSe (32.3%) (Fig. 5). It was difficult to ascertain which isoflavone form in the soybean extracts had a higher contribution towards the inhibition of α-glucosidase, primarily due to the difference in the amount of each isoflavone form within the various soybean extracts. Nevertheless, based on the results of the authentic isoflavone tests, it is assumed that isoflavone aglycones inhibit α-glucosidase more efficiently than isoflavone glucosides. In terms of the efficiency of soybean extract on α-glucosidase inhibition, blanching of soybean seemed to be more appropriate than cooking because of the higher rate of loss of isoflavone due to the intense treatment during the cooking procedure.
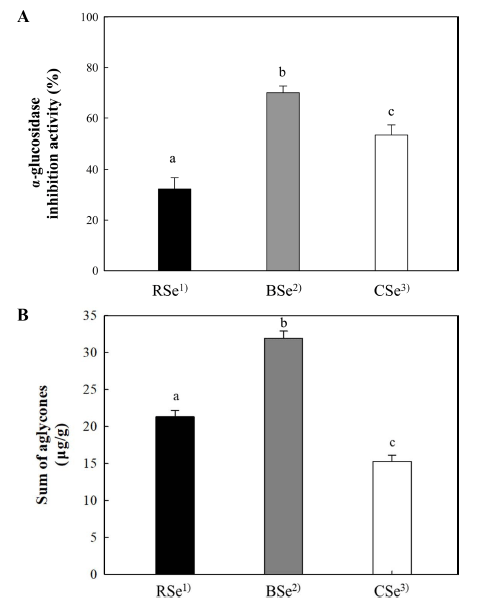