Introduction
The white-spotted flower chafer, Protaetia brevitarsis seulensis (Kolbe), is mainly distributed in East Asia (Yeo et al., 2013). Its life cycle consists of embryonic, larval, pupal, and adult stages. Of these, the larval stage is the longest. At this stage, the larvae supply and store energy for the rest of the lifecycle. Therefore, they are considered the most nutrient-rich (Kwak et al., 2014;Kim et al., 2020a). In 2016, the Ministry of Food and Drug Safety approved Protaetia brevitarsis seulensis larvae (PBSL) as a temporary food resource (Im et al., 2018;Choi et al., 2020). Furthermore, PBSL has also attracted attention for the development of novel materials that include not only functional foods but also feed, cosmetics, and pharmaceuticals (Lee et al., 2017;Choi et al., 2020). However, despite the numerous practical advantages, the scientific work on insects as food industrial materials is at a naive stage compared to studies on plants or animals because of its repulsive appearance (Yeo et al., 2013). Therefore, the research interests in developing protein-processing technologies capable of overcoming consumer prejudice against edible insects have increased (Mishyna et al., 2019;Kim et al., 2021).
It is well known that protein hydrolysates are easier to digest and more easily absorbed than intact proteins. Therefore, several studies have emphasized the need for developing health-functional foods based on protein hydrolysates from PBSL (Koopman et al., 2009;Kwak et al., 2014;Kim et al., 2020b). Furthermore, it has been shown that various processing methods, including physical treatments or biological treatments, can improve the food quality or the extraction yield of hydrolysates (Cha et al., 2010;Park et al., 2016). Of the several methods, the high-pressure extraction process in which the temperature and pressure are evenly transferred to the cell membrane owing to the pressure equalization has been shown to increase the degree of solubility of the cell membrane components and functional components, thereby improving the yield and quality of the extract even at low temperatures (Shouqin et al., 2004;Koo et al., 2007;Kim et al., 2015;Kim et al., 2017). Furthermore, recent studies have demonstrated the synergistic effect of the combination of high-pressure treatment and enzymatic hydrolysis on the increased yield of protein hydrolysates (Eisenmenger & Reyes-De-Corcuera, 2009;Kim et al., 2020b). It has also been shown that bioactive substances with low molecular weight obtained by high-pressure treatment maximize digestion and absorption in vivo (Lee et al., 2016). Therefore, it is expected that such substances could be useful to develop commercial products with different functional characteristics. However, the degree of decomposition and reaction may vary depending on various factors such as food type, characteristics, and temperature. For instance, at a pressure of 100 MPa or less, the enzymatic reaction rate varies depending on the denaturation of the substrate, stabilization of the enzyme structure, and enhancement of the enzyme-substrate binding affinity (Kim et al., 2018). Therefore, it is necessary to select the conditions of various process variables to obtain the desired outputs (Butz et al., 1994).
In a previous study, we investigated the production of protein hydrolysates from PBSL using different extraction methods, including high-pressure enzymatic hydrolysis (Kim et al., 2020b). The study focused on the effects of enzymes and showed that the extraction yield, protein content and yield, and amino acid profile of PBSL hydrolysates obtained by highpressure enzymatic extraction (HPE) increased 3.4 - 4.4 times compared to the other extraction method. Furthermore, Li et al. (2017) have shown that prolonged high-pressure treatment period could increase the contact between the solvent and solute contact for a longer duration, thereby increasing the extraction yield. Based on these findings, it was hypothesized that optimization of the HPE treatment period, another important factor in the HPE process, could maximize the production efficiency and quality characteristics of HPE hydrolysates from PBSL. To test this hypothesis, the present study was aimed to analyze the quality characteristics of protein hydrolysates prepared from PBSL during various HPE treatment periods to optimize the conditions for extraction of high-quality PBSL hydrolysates.
Materials and Methods
PBSL was obtained in powder form from Hongbaengyi of Maisan Mountain in Jinan, Jeonbuk, Korea, and stored in a frozen state. The obtained PBSL powder had a total protein content of 49.54±3.31 g/100 g and a moisture content of 5.15 ±0.02 g/100 g. Alcalase 2.4 L and Flavourzyme 1,000 L were purchased from Novo Nordisk (Bagsvaerd, Denmark), and Bromelain was purchased from Great Food (Bangkok, Thailand).
Gel Filtration Calibration Kit Low Molecular Weight (28- 4038-41; GE Healthcare, Uppsala, Sweden) was used for gel permeation chromatography (GPC) analysis, and 16 amino acids (glutamic acid, proline, aspartic acid, glycine, lysine, tyrosine, leucine, valine, serine, alanine, arginine, isoleucine, phenylalanine, threonine, histidine, and methionine) purchased from Sigma-Aldrich Chemical Co. (St. Louis, MO, USA) were used for amino acid analysis.
All other reagents were purchased from Sigma-Aldrich and JT Baker (Phillipsburg, NJ, USA).
PBSL powder (50 g) was mixed with 10 mM sodium phosphate buffer (pH 5.8) in a ratio of 1:14 (w/v), followed by the addition of Alcalase 2.4 L, Flavourzyme 1,000 L, and Bromelain each at 4% (w/w) relative to the substrate. The mixture was sealed and placed in a pressure vessel of the HPE equipment (TFS-2L, Innoway Co., Bucheon, Korea). The equipment had a capacity of 2 L, a pressure range up to 100 MPa, a temperature range from 25 to 79°C, and the treatment time could be adjusted from 1 min to 99 h. In this study, the pressure and temperature were set at 100 MPa and 50°C, respectively. HPE was performed for different treatment periods (0, 12, 18, 24, 30, and 36 h); at the end of each treatment period, the pressure was automatically released. The extracted sample was immediately removed and thermoinactivated at 100°C for 10 min. After centrifugation at 13,000 × g for 20 min, the supernatant was collected by filtration (Whatman filter paper No. 4). The supernatant was lyophilized and stored at -20°C as powdered "HPE hydrolysate" for subsequent analysis.
The extraction yield was calculated as the percentage of the weight of lyophilized HPE hydrolysates to the weight of PBSL used for extraction.
Protein yield was defined as the percentage of protein content in the HPE hydrolysates to the raw protein content in the PBSL. The protein content was measured using the Kjeldahl method by multiplying the total nitrogen content in the digested fraction after hydrolyzing HPE hydrolysates by the nitrogen-to-protein conversion factor (6.25) as described by Kim et al. (2020b).
The MWD of HPE hydrolysates was determined by the GPC method following the procedures described previously (Kim et al., 2020b). Briefly, the HPE hydrolysates were diluted to 2 mg/mL in HPLC water and filtered through a 0.45 μm polyvinylidene fluoride filter. The GPC was performed using an Agilent 1260 series HPLC system (Agilent Technologies, Santa Clara, CA, USA) equipped with a Superdex 7510/300 GL (17-5174-01, GE Healthcare) column. The mobile phase was 50 mM phosphate buffer (150 mM NaCl, pH 7.2). A flow rate of 0.5 mL/min and column temperature of 25°C were maintained for 70 min. The injection volume was 100 μL, and the wavelength of the UV detector was set at 280 nm.
Approximately 5 g of the HPE hydrolysate was hydrolyzed with 6 mol/L HCl at 105°C for 24 h under nitrogen. The hydrolysate was evaporated at 40°C under vacuum. The dried residue was then dissolved in 3-5 mL HCl (0.02 mol/L) and filtered through a 0.20 μm membrane filter (Life Science, MI, USA). Amino acid analysis was carried out using a Hitachi L- 8800 amino acid analyzer (Tokyo, Japan) with an ionexchange resin column (4.6 mm i.d. × 60 mm).
Results and Discussion
This study demonstrates the influence of HPE treatment period on the production of protein hydrolysates from PBSL by HPE. PBSL was hydrolyzed by HPE for 0, 12, 18, 24, 30, 36 h, wherein the other parameters of the HPE process were set at previously optimized conditions (Kim et al., 2020b).
The extract yield is a deciding parameter to evaluate the performance of the production process and the quality characteristics of the output (Nam, 2005). Therefore, we calculated and compared the yields of the HPE hydrolysate products prepared at various extraction times in the present study (Table 1). The results clearly illustrated the significant effect of HPE treatment period on the yields of HPE hydrolysates. The extraction yield was higher in samples subjected to longer HPE treatment. It was the highest at 30 h (40.09±0.03 g) and decreased thereafter. The protein yield also showed the highest values at 30 h; however, they were not significantly different from the contents estimated at 24 h. These results suggest that enzyme catalysis was accelerated under high pressure, increasing the dissolution of insoluble components and the elution of internal substances such as amino acids (Kim, 2009). Phhisit et al. (2017) reported that the addition of protease to corncobs and high-pressure treatment at 100 MPa, 50°C, for 10 min increased the enzyme efficiency by 180% compared to that of hot water extraction, resulting in the effective removal of hemicellulose. They also mentioned that the pressure energy changed the distribution of hydrophobic bonds and the cohesiveness of useful components in the plant cell wall, resulting in an increased extraction yield. In congruence, the synergistic effect between pressure and enzymatic hydrolysis is confirmed in this study, and the effect is demonstrated to be higher with increasing HPE treatment period. Furthermore, the tendency of the yield to increase with prolonged high pressure treatment was confirmed by Li et al. (2017) via analysis of the high-pressure extraction of astaxanthin from shrimp shells. Taken together, it can be inferred that in the HPE process, an appropriate HPE treatment period is crucial to maximizing the yield.
![]() |
The smaller molecular weight of a bioactive substance accelerates its acceptability as a value add to the functional food materials, as smaller compounds are more easily digested and absorbed in vivo. Therefore, the MWD of bioactive substances has been suggested as a key parameter to determine the quality characteristics of the product (Jin et al., 2009;Koopman et al., 2009). In this study, the GPC method, which provides information on MWD relatively quickly, was used (Holopainen et al., 1997). The analysis showed that the distribution of low molecular weight (< 1 kDa) components in the HPE hydrolysates increased as the HPE treatment period increased (Table 1 and Fig. 1). In particular, there was a change in the peaks at 150-1000 Da and less than 10 Da, and the change was clearly observed for 0-18 h, which may mean that the MWD of the HPE hydrolysates changes rapidly within 24 h. Initially (treatment time of 0 h), 51.89% of the total dry matter from the HPE hydrolysates had molecular weight < 1 kDa, which increased with increasing treatment period and was the highest at 36 h (67.32%); however, it was not significantly different from those obtained at 24 h (66.98%) and 30 h (67.14%).
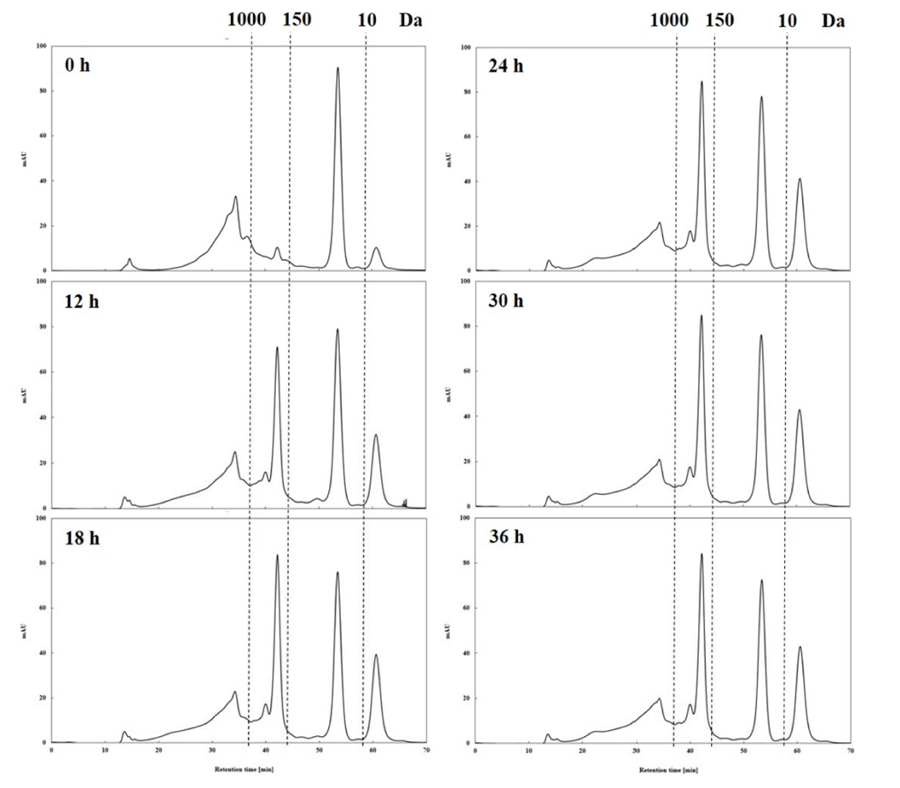
Furthermore, amino acids play an important role in determining the function and taste characteristics of food; thus, the composition and content of amino acids in protein hydrolysates are also important quality evaluation indices (Parsons et al., 1997;Kim et al., 2020b). The amino acid compositions of the HPE hydrolysates from PBSL according to HPE treatment period are shown in Table 2. Similar to other analysis results, the amino acid content was higher in HPE hydrolysates that were treated for longer. Both the total amino acid content and the essential amino acid content reached a significant peak at 30 h, showing favorable results not only in terms of content but also in composition. In the case of essential amino acids, the increased rate was highest for methionine, phenylalanine, isoleucine, leucine, and valine (up to 2, 1.8, 1.6, 1.5, and 1.4 times, respectively). Methionine reportedly prevents related diseases by regulating metabolic processes and the immune response (Martínez et al., 2017). Phenylalanine is an important precursor of many aromatic compounds required for normal body functions, contributing to blood vessel protection, mental stability, brain cell regeneration and hormonal activity (Harper, 1984). Isoleucine, leucine, and valine are branched-chain amino acids with important roles in human muscle activity as they are primarily oxidized in skeletal muscle and are used as an energy source by muscle cells (Sowers, 2009). Meanwhile, in the case of non-essential amino acids, tyrosine and serine exhibited a particularly high increase (up to 2.7, and 1.4 times, respectively). Serine promotes insulin secretion while lowering blood cholesterol and blood sugar levels (Kuo et al., 2020); whereas tyrosine contributes to memory improvement (Jongkees et al., 2017). In addition, glutamic acid content, which contributes to flavor characteristics, was increased, suggesting that the HPE treatment for an optimized duration could value add to HPE hydrolysates from PBSL in processed food, seasoned food, or feed material.
![]() |
Conclusion
HPE extraction of PBSL at 30 h maximized the enzymatic protein hydrolysis in PBSL and improved the quality characteristics, including total soluble solid content, extraction yield, total protein content, protein yield, MWD, and amino acid composition of the hydrolysate. These findings demonstrating an optimized condition for HPE could help develop strategies to improve the industrial use of functional food materials based on edible insects.