Introduction
The ginger root (Zingiber officinale Roscoe, Zingiberaceae) is one of the most heavily consumed spices and dietary substances in the world (Surh et al., 1998 ). Ginger contains a rich source of biologically active constituents including volatile or essential oil and the non-volatile pungent compound, oleoresin (Wang et al., 2009). The pungency of fresh ginger is primarily due to gingerols, which are a homologous series of phenols (Wohlmuth et al., 2005). Among the pungent compounds, gingerols are most abundant in fresh roots, and several gingerols with various chain lengths are present in ginger including 6-, 8-, and 10-gingerol (Fig. 1). 6-Gingerol is considered to be the major compound of ginger and has been found to exert various pharmacological and physiological effects including analgesic, antipyretic, gastroprotective, cardiotonic, and antihepatotoxic activities (Suekawa et al., 1984). The pungency of dry ginger mainly results from shogaols, which are the dehydrated forms of 6-gingerol and the form including 6-shogaol. Shogaols are found in dried and thermally treated roots, accordingly, thermal dehydration has been proposed for the conversion of gingerols into shogaols (Chrubasik et al., 2005). Among shogaols, 6-shogaol is the most abundant and has anti-tumor and anti-gastric cancer effects (Zhang et al., 2008).
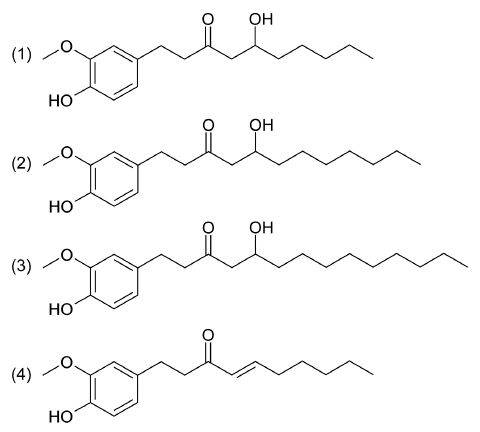
Herbal medicines have been used worldwide for thousands of years, particularly in Asian countries. According to ancient texts such as Shikryoboncho from the Dang dynasty in China, the thermal processing of herbs significantly influences its pharmacological and toxicological properties. Typically, processing of herbs consists of nine cycles of steaming and drying. Indeed, this process, which is known as kujeungkupo in Asian countries, has traditionally been applied to Thea sinensis, Polygonatum odoratum, and Rehmannia gluyinosa to increase their biofunctional properties (Howard & Fazio, 1980). In Korea, black ginseng has been produced using this traditional method to increase the yield of pharmaceutical compounds. However, there are some problems associated with this method, including difficulties of controlling the process properly and the chance of forming potentially hazardous compounds such as benzo(a)pyrene (BaP), which is a member of the polycyclic aromatic hydrocarbons (PAHs) and recognized as a carcinogen (Phillips, 1999). BaP is mainly formed during processing or cooking techniques such as smoking, roasting, baking and frying. Recently, some countries, including the United States and the European Union, established a daily allowance of maximum level of BaP in different foodstuffs (Mensor et al., 2001). This study was conducted to develop a well-controlled manufacturing method for black ginger that removed the possibility of the formation of BaP and enhanced the formation of shogaol during processing. In addition, the physicochemical and biofunctional properties of black ginger were analyzed.
Materials and Methods
Rhizomes of fresh ginger (Zingiber officinale Roscoe) harvested in Korea were obtained from a local food market. 6-, 8-, and 10-gingerol and 6-shogaol were obtained from ChromaDex Inc. (Santa Ana, CA, USA). HPLC-grade methanol, acetonitrile and ethyl acetate were obtained from Fisher Scientific Co. (Fair Lawn, NJ, USA). All other chemicals were purchased from Sigma-Aldrich Co. (St. Louis, MO, USA) unless otherwise indicated.
A 3.0 kg of fresh rhizomes of ginger were washed with distilled water, and the whole body was then cut into approximately regular size. And then, 300 g of individual sample were placed in a steaming jar and then air-dried at room temperature. Black ginger was produced using a wellcontrolled autoclaving method. Briefly, ginger was steamed at 121°C and 15 lb/in2 for 30 min, after which it was dried at 60°C for 12 h. The steaming and drying steps were repeated nine times, with the sample being collected at each step. The collected sample was ground to a fine powder through a 100- mesh sieve and kept frozen at -20°C until subsequent analysis.
The difference in the color of the ginger powder was analyzed at each step using a digital color difference meter (UltraScan PRO D65, HunterLab, Reston, VA, USA). All samples were measured three times and the average values were expressed as L* (lightness), a* (redness), b* (yellowness) and ΔE (overall color difference). The L*, a* and b* values of a standard white plate were 97.52, -0.06 and 0.03, respectively.
6-, 8-, 10-Gingerol and 6-shogaol were used as the standards in this study. These compounds were dried over silica gel for 3 h under vacuum, after which 10.0 mg of 6-, 8-, 10-gingerol and 6-shogaol were weighed and transferred to screw-capped glass centrifuge tubes (16 mm×125 mm). Next, 10 mL of HPLC-grade methanol were added to each standard to produce a stock solution with a final concentration of 1.0 mg/mL. Serial dilutions of the standard were then made to produce 10, 25, 50, 100, and 250.0 μg/mL working standards, and stored at 4ºC until used. The chemical structures of 6-, 8-, 10-gingerol and 6-shogaol were shown in Fig. 1.
A 10 g sample of each ginger powder was mixed with 100 mL of ethyl acetate, and then extracted on an Eberbach shaker (LS203L, LSTECH, Seoul, Korea) at 180 rpm for 24 h at room temperature. And then, each sample was centrifuged at 17,500×g (J2-MC, Beckman Coulter, Inc., Brea, CA, USA) at 4ºC for 10 min. The supernatant was then evaporated in a vacuum rotary evaporator (N1000, Eyela, Tokyo, Japan), and the residue was dissolved in 1 mL of methanol, filtered through a syringe filter (PTFE, 0.45 μm) and subjected to HPLC analysis.
Compositions of ginger compound were analyzed using an HPLC system (Alltech Series 627 liquid chromatography, Alltech Associates, Deerfield, USA). Chromatographic separation was conducted at 40ºC on a C18 column (4.6 mm×250 mm×5 μm; CAPCELL PAK, Shiseido, Tokyo, JAPAN) with 0.1% trifluoroacetic acid-methyl alcohol (34:66, v/v) as the mobile phase. The HPLC operating parameters were as follows: injection volume, 20 μL; column flow rate, 1.0 mL/min; chromatographic run time, 50 min; UV spectra recording, 282 nm. Each peak of 6-, 8-, 10- gingerol and 6-shogaol was identified based on the retention time of the standard and further confirmed by comparing their UV spectra to those of the individual standards. The standard curves were obtained from 10 to 250 μg/mL for 6-, 8-, 10-gingerol and 6-shogaol.
A 1 g of sample was extracted three times with 10 mL of nhexane for 1 h. After centrifugation at 17,500×g, the three extracts were combined and 1 mL of 3-methylcholanthrene (10 ng/mL) was added as an internal standard. The combined extract was washed with 50 mL of distilled water by shaking in a separating funnel, after which the hexane phase was collected, dried and concentrated with 15 g of anhydrous sodium sulfate in a vacuum rotary evaporator (N1000, Eyela, Tokyo, Japan). The dried residue was diluted in 2 mL of hexane and purified using a florisil SPE cartridge (Supelclean LC-Florisil SPE, Sigma-Aldrich, St. Louis, MO, USA) that was equilibrated with 10 mL of dichloromethane and 20 mL of hexane. Analyte (2 mL) were applied to a SPE cartridge (5 g) and eluted with hexane and hexane/dichloromethane (70:30, v/v). 5 mL of hexane were discharged and 15 mL of hexane/dichloromerhane were then used to elute the PAH fraction. The flow rate was adjusted to about one drop per second and the collected fraction was concentrated in a vacuum rotary evaporator at 40ºC and dried under a flow of nitrogen. The dissolved residue in 1 mL of acetonitrile and filtered through a syringe filter (PTFE, 0.45 μm) before injection into a HPLC.
BaP of black ginger powder was analyzed using a HPLC system (Gilson 305 series liquid chromatography, Gilson Inc., Middleton, WI, USA) equipped with a dual pump. The detector was a programmable spectrofluormeter (ProStar, Varian, Tokyo, Japan) set at optimized excitation (Ex) and emission (Em) wavelengths, which were Ex 294 nm and Em 404 nm. For separation, a C18 column (4.6 mm×250 mm×5 μm; CAPCELL PAK, Shiseido, Tokyo, Japan) was used. The mobile phase consisted of acetonitile-distilled water (80:20, v/ v) at a flow rate of 1 mL/min (isocratic) for 50 min.
Tyrosinase inhibition assay was conducted using mushroom tyrosinase. Briefly, 10 μL of L-tyrosine (1.5 mM), 10 μL of 0.1 M phosphate buffer (pH 7.4) and 10 μL of various black ginger extracts (6.66 mg/mL) were added to a 96- well plate. Arbutin was used as positive control. The plate was then preincubated at 37ºC for 5 min, after which 10 μL of tyrosinase (200 U/mL) were added. The microplates were incubated for an additional 10 min at 37ºC, and the optical densities were determined at 490 nm using an ELISA reader (Synergy HT, BioTek Insruments Inc., Winooski, VT, USA).
Free radical scavenging potentials of each sample were evaluated using DPPH solution and the antiradical activity was defined as the amount of antioxidant necessary to decrease the initial DPPH concentration by 50% of the efficient concentration (EC50) as described by Mensor et al. (2001). A 1 g of each sample mixed with 10 mL of ethyl alcohol were sonicated for 1 h (Power sonic 520, Whashin, Eiwang, Korea) and subsequently centrifuged at 17,500×g at 4ºC for 10 min. The supernatant was evaporated in a vacuum rotary evaporator and diluted to final concentrations of 10, 20, 40, 60, 80 and 100 μg/mL in ethyl alcohol. Next, 1.0 mL of 0.3 mM DPPH solution in ethyl alcohol was added to 2.5 mL of samples containing different concentrations of black ginger extract. Each sample was kept at room temperature in the dark for 30 min, and the absorbance value was then measured at 518 nm. The antioxidant activity (AA) was determined using the following formula:
AA (%) = 100 - ((Abssample - Absblank) × 100)/Abscontrol
The blank consisted of 2.5 mL of extract solution with 1.0 mL of ethyl alcohol and 2.5 mL of ethyl alcohol with 1.0 mL of 0.3 mM DPPH solution was used as a negative control. An antioxidant like vitamin E, 6-hydroxy-2,5,7,8-tetramethylchroman- 2-carboxylic acid (Trolox, Sigma-Aldrich, St. Louis, MO, USA), was used as a positive control. The EC50 values were calculated by linear regression of plots in which the abscissa represented the concentration of black ginger extracts and the average percentage of antioxidant activity. All experiments were conducted in triplicate.
Results and Discussion
Fresh ginger was processed by conducting steaming under autoclaving conditions (121ºC, 15 lb/in2 for 30 min) and drying at 60ºC for 12 h for nine times in a modified version of the traditional kujeungkupo method (Fig. 2). The thermal processing was applied to sterilize heat-resistant microorganisms and increase the amounts of specific biofunctional products (Kim et al., 1981).
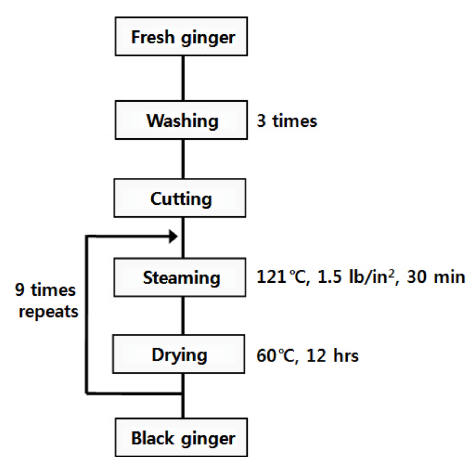
During the manufacture of black ginger, the lightness (L* value) and yellowness (b* value) decreased from 85.65±0.33 and 26.99±0.20 in the non-treated ginger to 56.91±0.25 and 16.69±0.06 in the ninth-treated ginger, respectively (Table 1). Conversely, the redness (a* value) increased from -1.51±0.03 to 7.34±0.08 in the eighth-treated ginger and decreased to 7.21±0.04 in the ninth-treated ginger. Moreover, the values of the overall color difference (ΔE) increased from 29.49±0.05 to 44.49±0.25. During the steaming and drying steps, the pale yellow color of fresh ginger and its powder became dark brown (Fig. 3), primarily due to polymerization of the sugars and amino acids with heat treatment. The browning of the thermal processed product has been shown to have increased antioxidant activity (Ojewole, 2006).
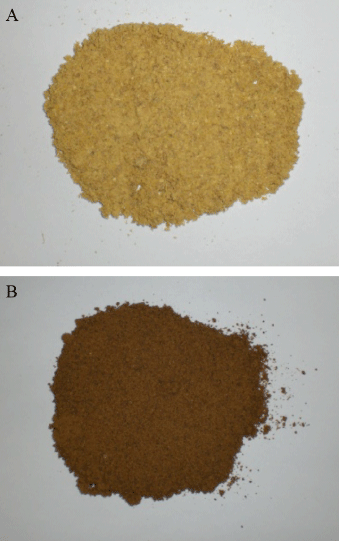
Gingerols and shogaol, which are typical ginger-derived components, have potent anti-cancer and anti-inflammatory activities (Bhattarai et al., 2001). Therefore, their compositions should be considered when ginger products are being developed for pharmaceutical applications. 6-, 8-, 10-gingerol and 6-shogaol were quantitatively analyzed by HPLC using external standards and their contents are shown in Table 2. During processing, the contents of 6-gingerol decreased from 3.257±0.067 mg/g in the non-treated ginger to 0.567±0.036 mg/g in the ninth-treated ginger, whereas the contents of 6- shogaol increased from 1.299±0.050 mg/g in the non-treated ginger to 2.999±0.089 mg/g in the sixth-treated ginger and decreased to 2.099±0.039 in the ninth-treated ginger. The contents of 10-gingerol decreased slightly from 1.106±0.125 mg/g to 0.806±0.026 mg/g in the ninth-treated ginger. Unlike 6- and 10-gingerol, the contents of 8-gingerol changed slightly, with values between 0.916±0.005 mg/g and 1.106±0.005 mg/g being observed during processing. The results showed that 6- gingerol was thermally dehydrated into 6-shogaol (Fig. 4). Bhattarai et al. (2001) reported that the conversion of 6- gingerol into 6-shogaol was reversible under acidic conditions and dependent on pH and temperature. However, in this study, the reaction was not found to be reversible (Fig. 4). The decrease in 6-shogaol observed after the sixth treatment might have been due to the polymerization of shogaol under high temperatures (Hu et al., 2009).
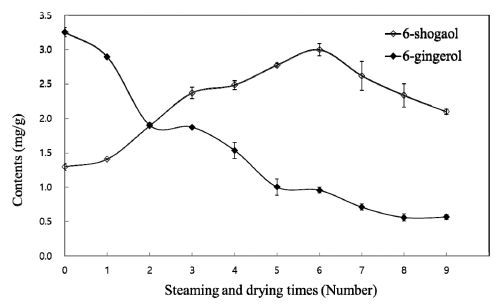
Polycyclic aromatic hydrocarbons (PAHs) are formed for various reasons and produced during the combustion of any carbohydrates. In food stuffs, PAHs are generated by improper heat-treatment during processing or cooking. Among PAHs, BaP has been categorized as group 1 (carcinogenic to human) by the IARC (International Agency for Research on Cancer) (Tfouni et al., 2007). No BaPs were identified in the manufactured black gingers. Under these manufacturing conditions, moisture was kept, which prevented ginger from combusting and did not allow it to produce potentially hazardous compounds. As a result, the autoclaving method used in this study was suitable for the manufacture of black ginger without producing BaP.
Tyrosinase is a rate-limiting enzyme in the melanogenesis of a living body that catalyzes the initial step in the formation of the pigment melanin from tyrosine. In the present study, the black ginger extract showed potent tyrosinase inhibitory activity (Fig. 5). The tyrosinase inhibitory activity of black ginger extracts were increased from 43.42±11.45% in the nontreated ginger to 100% in the sixth-treated ginger, while they decreased to 51.98±7.36% in the ninth-treated ginger. This tendency showed a similar pattern to the changes in the 6- shogaol contents (Fig. 4), suggesting the possibility of a whitening effect of the black ginger produced in this study and that 6-shogaol was the functional component of the black ginger.
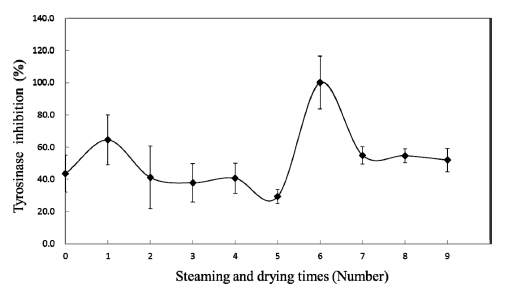
The antioxidative activities of the processed gingers were evaluated using a DPPH scavenging acidity assay. The EC50 value of ginger extract in each processing step is shown in Table 3. The values were between 25.083±0.050 μg/mL and 26.406±0.871 μg/mL when the value of Trolox as a positive control was 45.186±0.196 μg/mL. These results showed that antioxidative activities of the processed gingers were not influenced by the autoclaving conditions. Dugasani et al. (2010) reported that the relative scavenging potentials occurred in the order of 6-shogaol > 10-gingerol > 8-gingerol > 6- gingerol. Unlike their results, the antioxidant activities of black gingers in this study were retained despite the conversion of 6- gingerol of ginger into 6-shogaol during the steaming and drying processes. These findings indicate that some factors other than 6-shogaol influenced the antioxidative effect of the product.